1951
The Founding of Project Sherwood
Interest in controlled thermonuclear fusion began before the start of World War II, with researchers in the United States, United Kingdom and Soviet Union performing early experiments with plasma on a device known as "pinch" – basically a fluorescent light tube filled with hydrogen gas that would be ionized with a large electrical current.
This interest would grow throughout the war, when scientists working on the Manhattan Project began to consider the application of fusion in weapons and beyond.
In 1951, the U.S. Atomic Energy Commission (AEC) established Project Sherwood, a secret program to investigate the feasibility of using a controlled fusion reaction to generate power. It was originally estimated that spending about $1 million a year over 3-4 years would be sufficient to determine whether a high-temperature plasma could be confined by a magnetic field.
Classified Project Sherwood teams were initially established at Los Alamos National Laboratory, Princeton University, and the University of California's Radiation Laboratory, which would later become Lawrence Livermore National Laboratory, to investigate different configurations of fusion devices. The main configurations studied were magnetic mirrors, which were like the pinch devices, but with strong magnetic fields at either end that "bounced" the plasma into a middle confinement field, and stellarators, which bent the tube into a circle, creating a twisting, doughnut-shaped torus of plasma.
In 1955, at the first Geneva Conference on the Peaceful Uses of Atomic Energy in Swizterland, the delegation from India publicly announced their country was pursuing a national program in controlled magnetic fusion research. Soon, other countries revealed their own fusion research programs, including the U.S. and Soviet Union, and by 1958, at the second Geneva Conference, fusion research was largely declassified and the nations involved were displaying their work to the public. Project Sherwood was dissolved, and what remained became part of the AEC's Controlled Thermonuclear Research program.
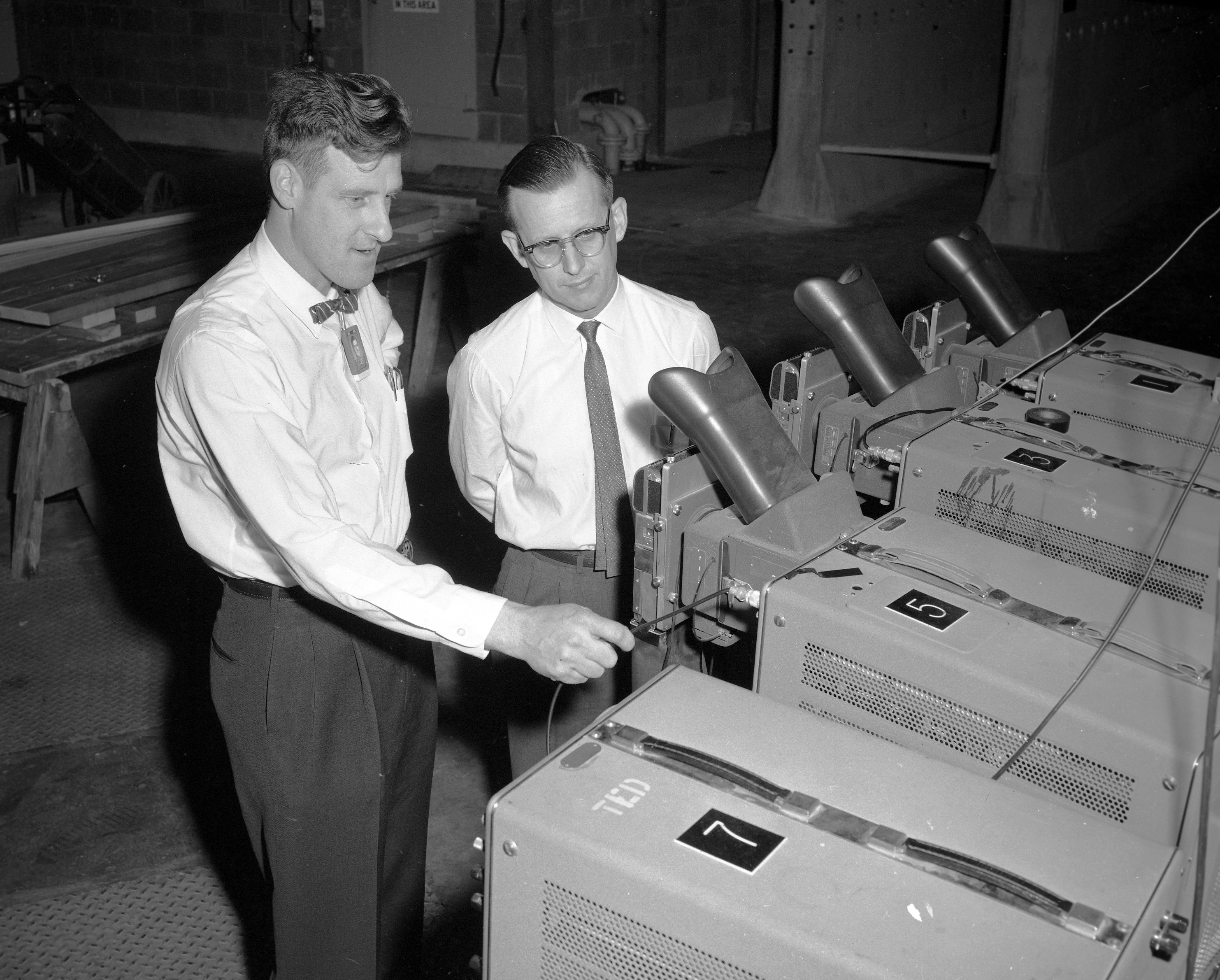
1952
The Origins of Fusion at ORNL
E.D. Shipley, who served as the director of research for Y-12, became ORNL’s associate director for research after the transfer of Y-12’s research divisions to ORNL.
In 1952, Shipley formed a group within the Electronuclear Division to begin theoretical studies of controlled thermonuclear fusion under Project Sherwood. The first group members included Mozelle Rankin (later Bell), Rodger Neidigh, Clarence Blue, John Luce, and Harry Hoy.
Al Simon, a theorist at ORNL, delivered a series of lectures about the physics of plasma confinement, while Rodger Neidigh developed an experiment that determined that the magnetic fields needed to confine a plasma would not have to be as powerful as previously thought.
The group's initial work focused on repurposing Calutrons, the electromagnetic isotope separation devices that had been used to enrich uranium at Y-12 throughout World War II. John Luce, who came from California to help design and build much of the equipment at Y-12, originally modified the Calutron ion source to support Lawrence Livermore's magnetic mirror program.
Luce's design used Calutron ion sources, enclosed in large tanks that acted like vacuum chambers, that injected molecular deuterium, an isotope of hydrogen, into a magnetic field. He theorized that the molecules could be dissociated, or fragmented, into ions using a powerful arc discharge, like that from a welding machine, and the resulting ions would create a high-temperature, high-density plasma needed for fusion. This design would be the genesis for the first large-scale confinement experiment at ORNL, the Direct Current Experiment.
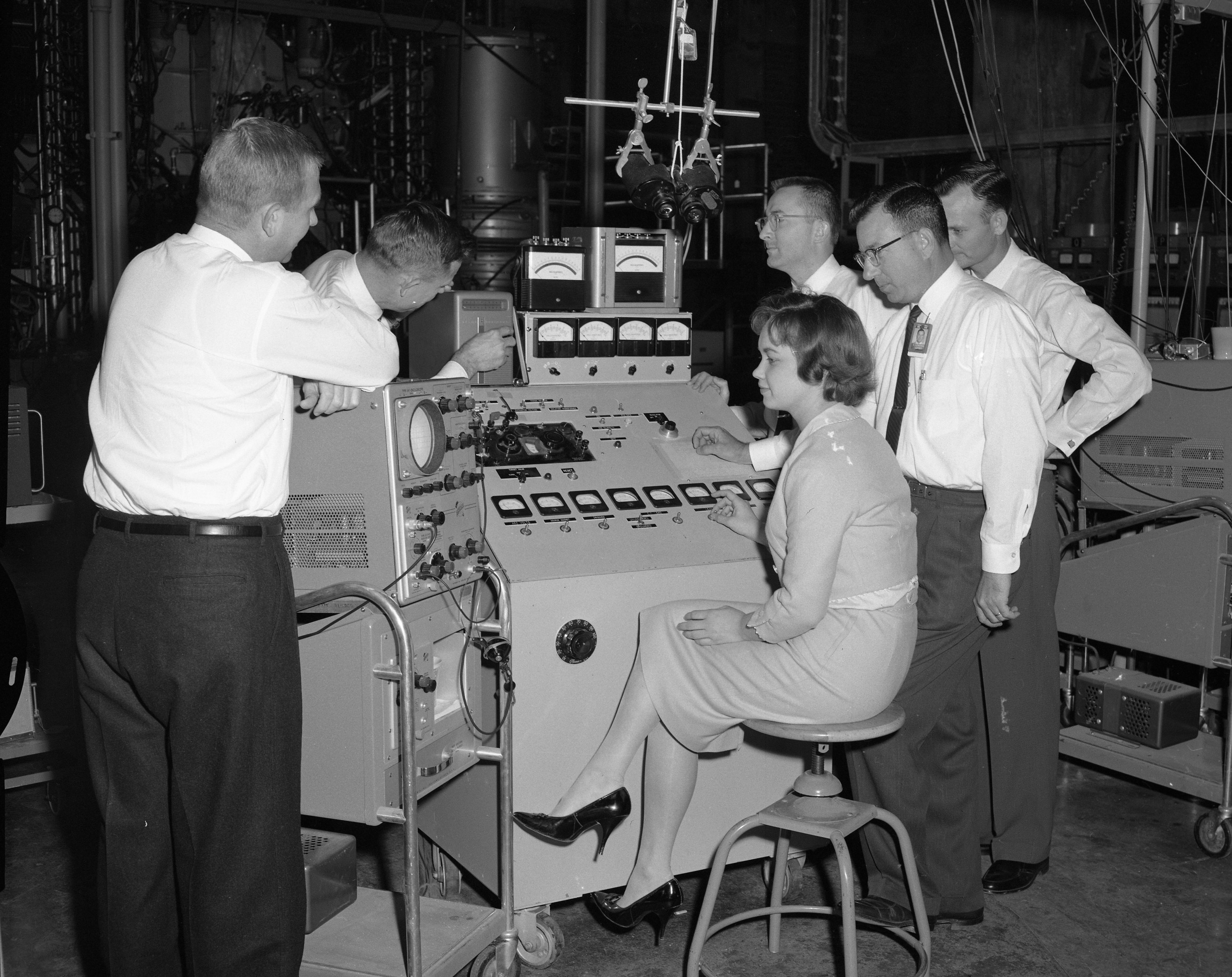
1956
The Direct Current Experiment (DCX)
The Direct Current Experiment, or DCX, was the first fusion confinement experiment at ORNL.
Work on DCX began in the fall of 1956 under the direction of E.D. Shipley and P.R. Bell. The device consisted of two adjacent Calutron tanks with holes bored between them, a pair of coils to generate a magnetic field, and a modified Calutron ion source for injecting molecular hydrogen. Two graphite electrodes hooked up to a large generator created a carbon arc several feet long, with currents of several hundred amperes, that would dissociate the beam of molecules into ions. The energetic protons resulting from the dissociation would be captured in a ring along the magnetic field. A small hopper filled with tungsten powder was placed above the magnetic field that would shake out a few particles when a button was pressed. The falling particles would then glow and show the shape of the otherwise invisible hydrogen ring as they passed through.
The goal of the first DCX device was to investigate the phenomena of burnout and exponentiation. Theoretical studies showed that the injected neutral molecules could be ionized as soon as they entered the plasma region, and the remaining non-ionized molecules would be "burned out" and ejected, which would reduce energy loss and create a hotter, more stable plasma. The trapped protons would then be able to dissociate incoming molecular ions themselves without the arc discharge, increasing proton density, which would in turn increase the dissociation rate, and the density would grow exponentially further.
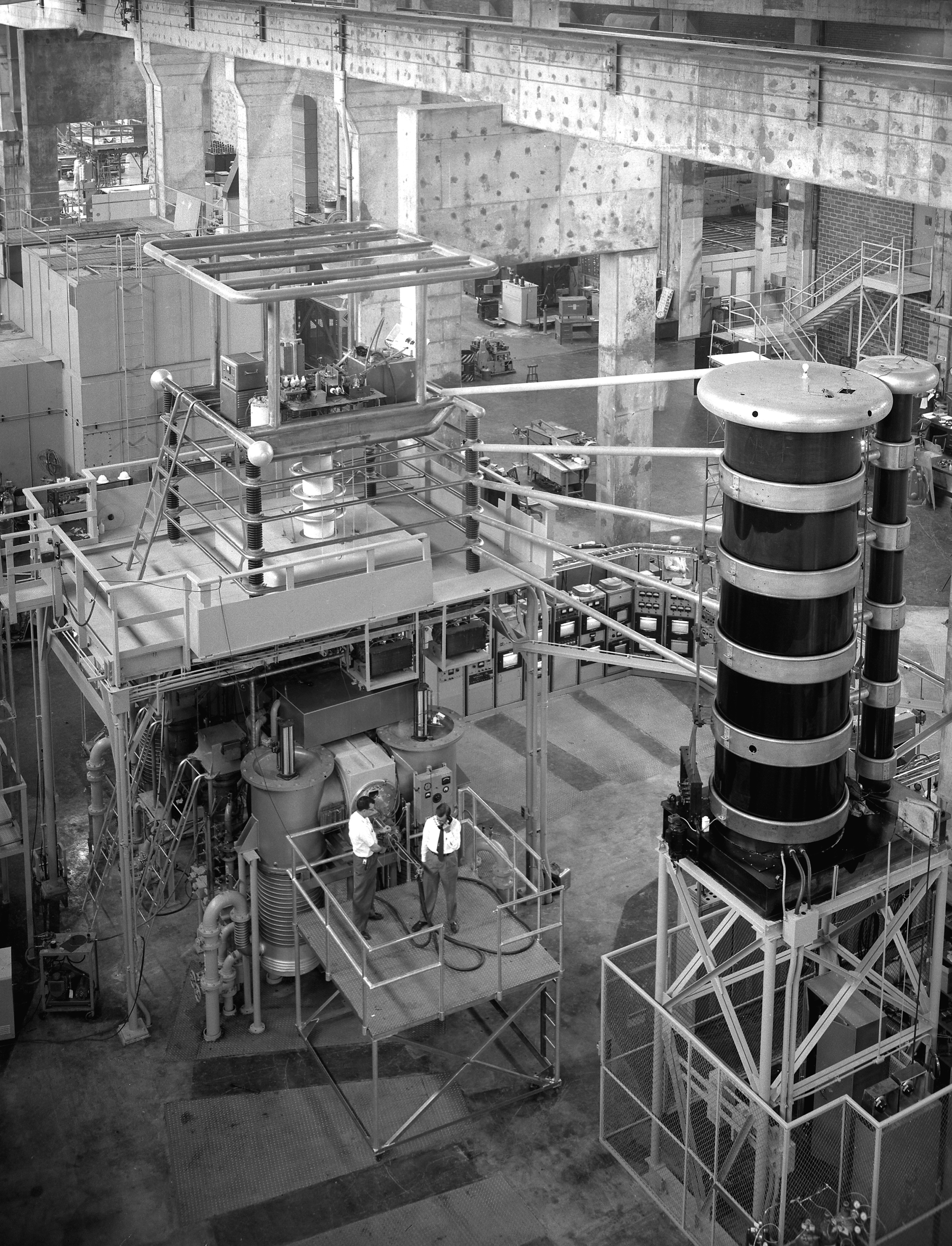
1958
DCX in Geneva
The Direct Current Experiment was publicly displayed for the first time in 1958 at the second Geneva Conference on the Peaceful Uses of Atomic Energy in Switzerland, also known at the "Atoms for Peace Conference." For two weeks, more than 5,000 scientists, government officials, and spectators from 67 countries convened to witness the progress of nuclear science and the debut of many new fusion technologies.
A contingent of ORNL scientists spent several months in Switzerland in the run-up to the event to assemble and test the exhibit. Two identical DCX devices were built, so one could always be on display while the other one was being maintained. The exhibition was popular, with crowds of people lined up to look through the DCX viewing port and see the glowing ring of plasma accumulated within.
There was one minor incident, however, owed to a cross-cultural misunderstanding: The pumps used to extract air from the vacuum chambers routinely emitted clouds of black smoke, which the visiting Oak Ridgers considered normal. The local Swiss firefighters, who were already on high alert with the amount of high energy equipment in the wooden exhibition hall, were not aware of this and rushed to the scene, demanding (in French) to know the source of the smoke from the confused researchers, which took quite some time to explain.
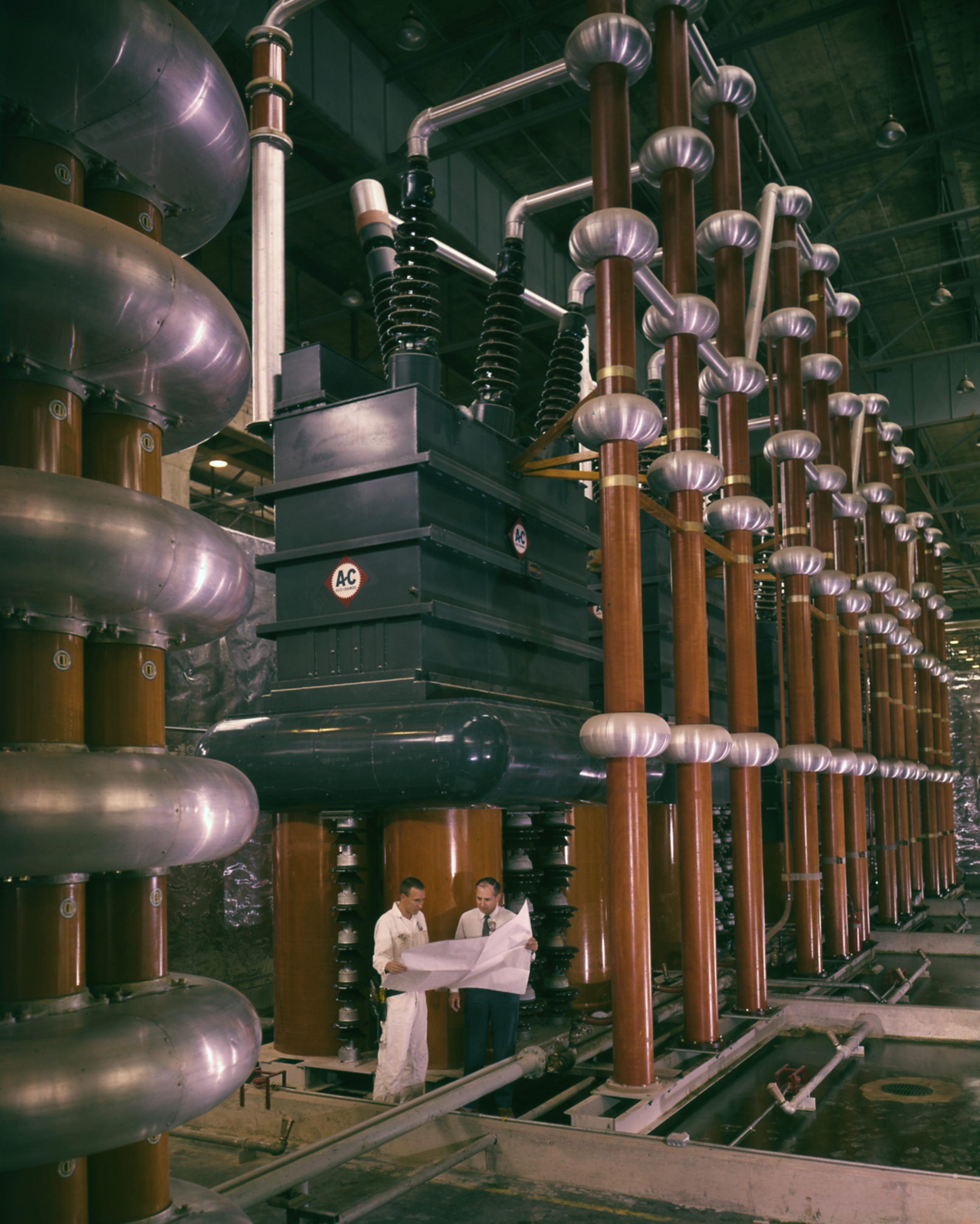
1959
Physics Test Facility (PTF) and Long Solenoid Facility
In 1959, the Project Sherwood group at Oak Ridge became the Thermonuclear Project under Art Snell, one of ORNL's associate directors. The group was operating out of Building 9204-3 on the Y-12 campus, where they had more room and access to high-power generators. Along with DCX, the building also housed the Physics Test Facility (PTF) and the Long Solenoid Facility.
The PTF was one of the earliest radiofrequency heating experiments at the lab. It was built to inject annular, or ring-shaped, plasmas using crossed electric and magnetic fields. Later in the 1960s, microwave power was also applied to the the PTF, pioneering the study of electron cyclotron heating, or ECH. ECH is the process of heating a fusion plasma using microwaves that match the natural frequency of electrons in a magnetic field, and is a vital part of the start-up process, heating, and instability control in plasmas.
The Long Solenoid Facility was built to develop vacuum arcs for other fusion experiments, and would later be used to develop the deuterium and lithium arcs for DCX's successor, DCX-2. The main magnet coils in the Long Solenoid Facility consumed so much power that a new method of providing cooling water to the coils had to be developed, as the old methods proved inadequate. This new water-cooling method would later be incorporated into other fusion experiments, including the ELMO Bumpy Torus.
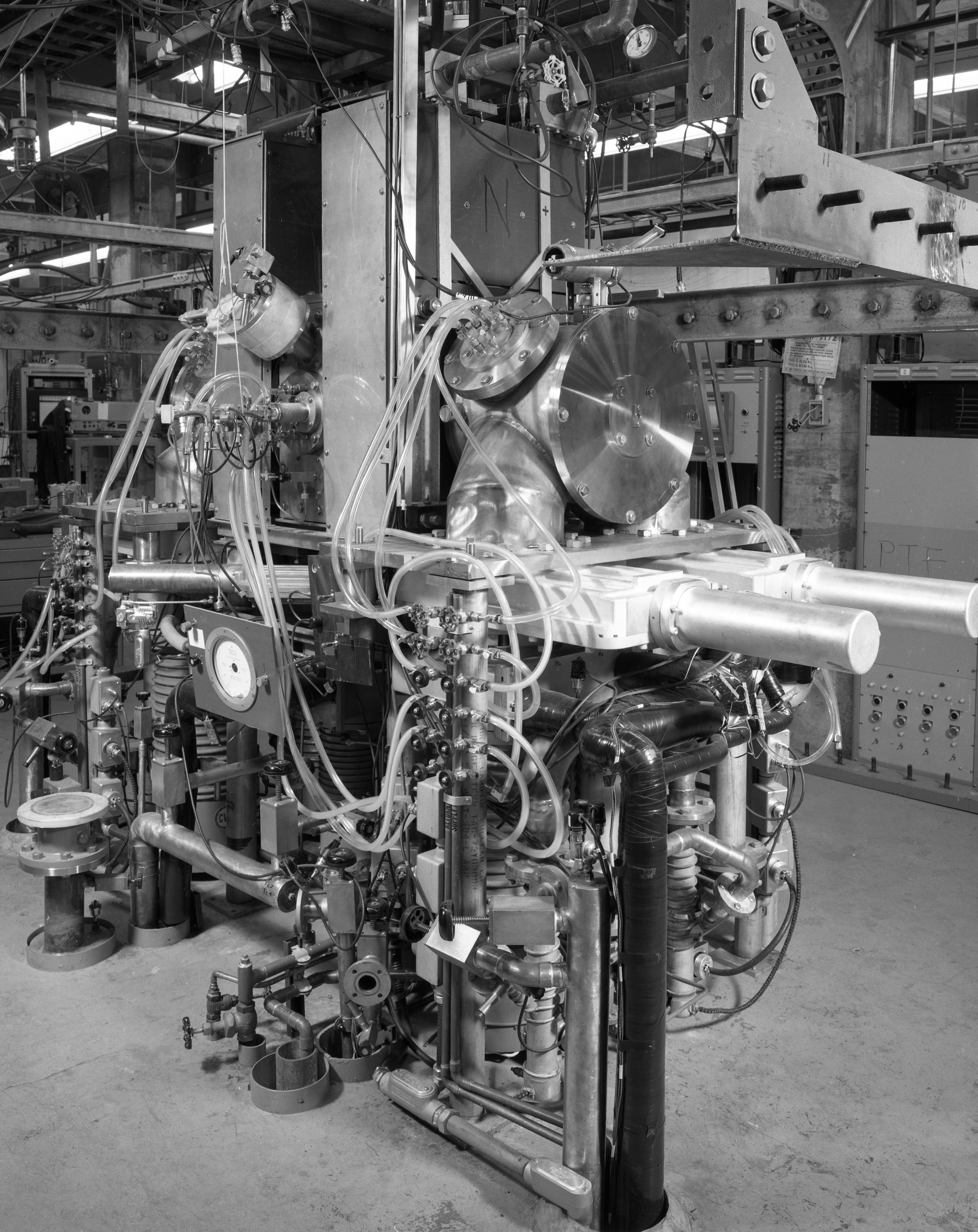
1960
DCX-2, DCX-EPB, and INTEREM
The DCX device, which would later be dubbed DCX-1, was always seen as a "first step" machine. Once it was clear that DCX-1 was not going to achieve burnout or exponentiation, plans were made for its successor device in 1960.
Two designs were proposed, DCX-2 and DCX-EPB, but only one could be built. Both designs were fairly similar, with long central containment sections and strong magnetic fields at either end to act as magnetic mirrors. Ultimately, DCX-2 was chosen, as it could produce much higher beam currents and could accelerate ions very quickly using an accelerator tube only about a foot long. DCX-2 performed its first experiments in 1962.
While DCX-EPB was not chosen for the main experiment, a smaller, low-voltage version was built at ORNL by John Luce, called DCX-EPA, to dissociate different forms of molecular hydrogen with a more advanced accelerator. DCX-EPA was later converted into a simple mirror called INTEREM, which recycled one of the vacuum chambers from the 1958 Geneva exhibit, and was used to study multiple mirror concepts that culminated in the Mirror Fusion Test Facility-B at Lawrence Livermore.
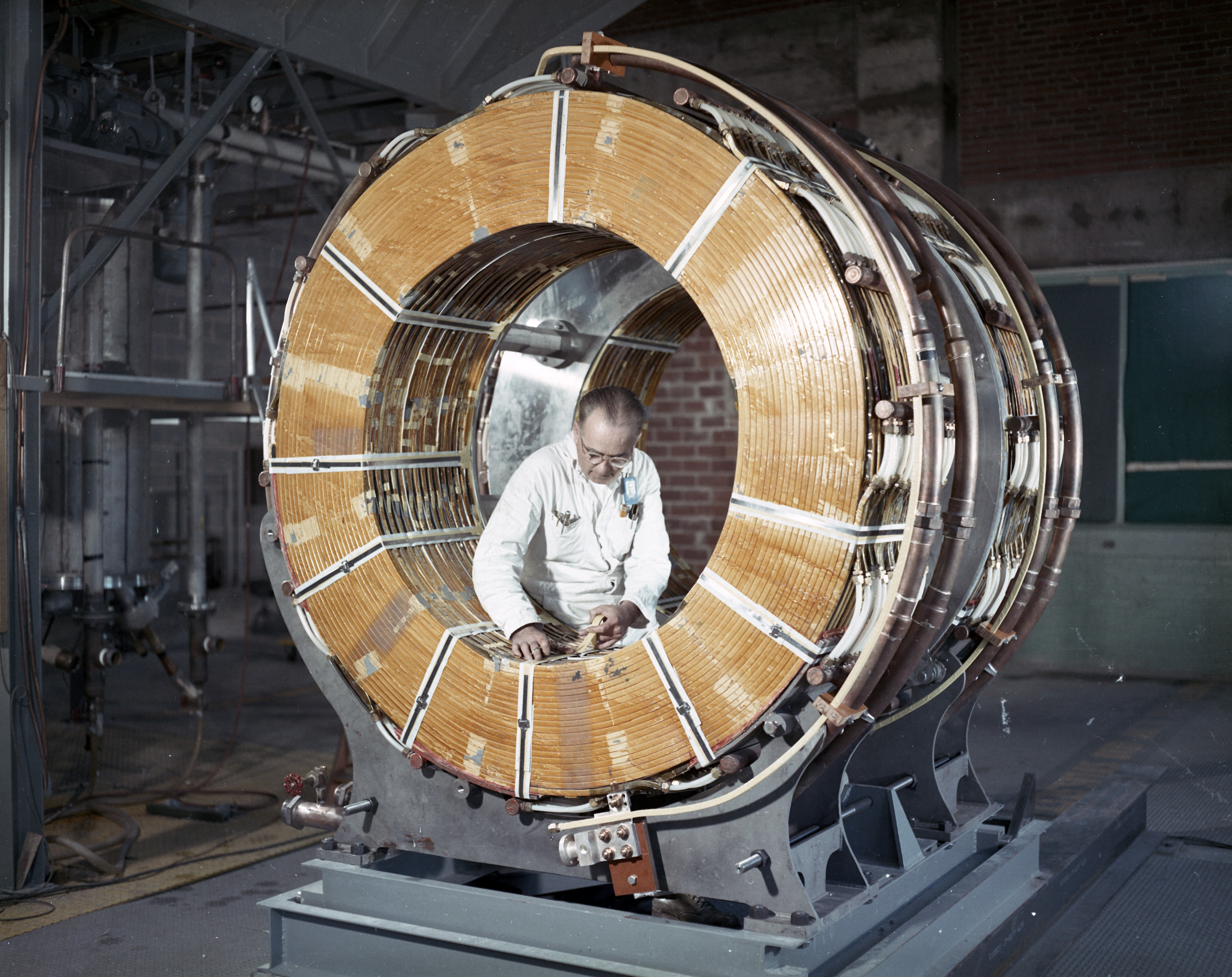
1969
Injection into Microwave Plasmas (IMP)
The Injection into Microwave Plasmas (IMP) device was a stabilized mirror machine in which beams of neutral particles were injected into a plasma formed by microwaves. IMP was the first ORNL experiment to use a neutral particle beam specifically to heat a plasma.
It was also one of the first fusion experiments to use superconducting coils, specifically ones made of niobium-titanium and niobium-tin, which are now used in accelerators and fusion experiments around the world, including ITER.
Due to the strong magnetic forces generated by the coils and concerns about weld strength, the coil cases were extensively machined from large individual ingots of stainless steel over the course of months.
IMP operated until 1972 and was the last linear mirror device built at ORNL.
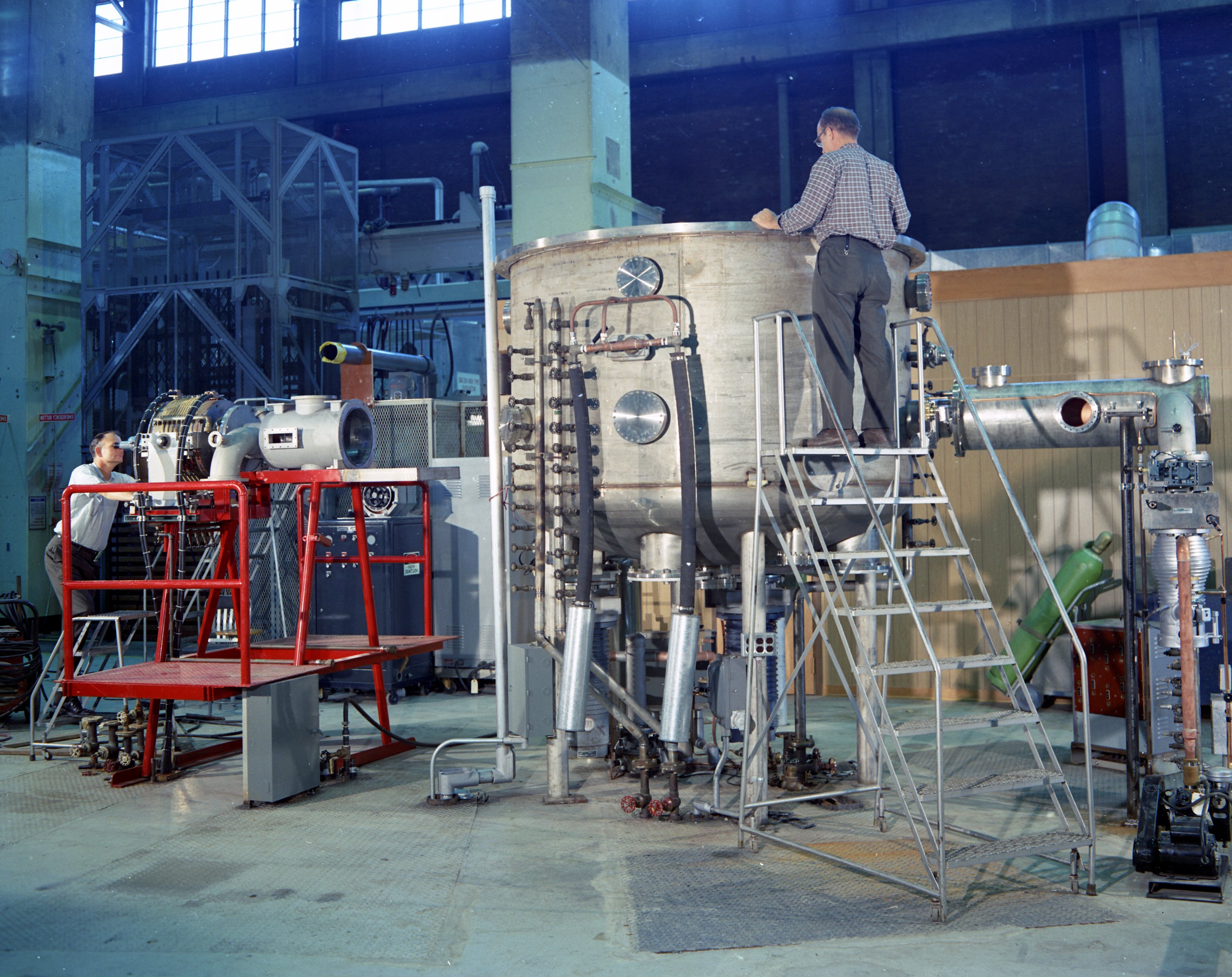
1972
From Mirrors to Toroids
While the U.S. mirror program would continue into the 1980s, the closure of the IMP facility in 1972 marked ORNL’s transition away from mirror research towards toroidal, or circular, devices, like stellarators and tokamaks.
Though they were phased out for more promising designs, smaller-scale mirror devices built in the 1960s and early 1970s, like the Canted Mirror Facility, were important for developing the concepts later used in larger devices.
The Canted Mirror Facility (1970-71) helped define the appropriate angle for the mirrors in the ELMO Bumpy Torus, using mirror coils repurposed from the Long Solenoid Facility mounted on pivots to simulate a section of a torus.
The steel vacuum chamber of the device tended to absorb microwaves and become very hot, so to keep it cool, it was wrapped in cheesecloth and soaked in cold water during operation. Once it had fulfilled its purpose, the device was given a second life at the University of Wisconsin, where it continued to be used for many years.
1971
ORMAK
Following the termination of the DCX-2 project in 1969, fusion resources at ORNL were directed toward toroidal and tokamak research and the design of ORMAK, the lab's first tokamak experiment.
ORMAK, which was completed in 1971 and operated until 1976, was one of the first tokamaks equipped with neutral beam heating technology and the first to demonstrate the heating of electrons by neutral beams. It was also the first tokamak to test pellet injection, where solid pellets of frozen hydrogen isotopes are shot at high speed into the plasma to fuel, regulate, and even terminate the reaction.
ORMAK's coils operated inside a large "bell jar"-like vacuum tank cooled with liquid nitrogen, which permitted high current and high magnetic fields, but made it hard to install or modify diagnostics. It had a gold-plated liner and tungsten components, both of which served as sources of heavy element impurities in the plasma and demonstrated that plasma impurities could cause extremely large losses of electromagnetic radiation.
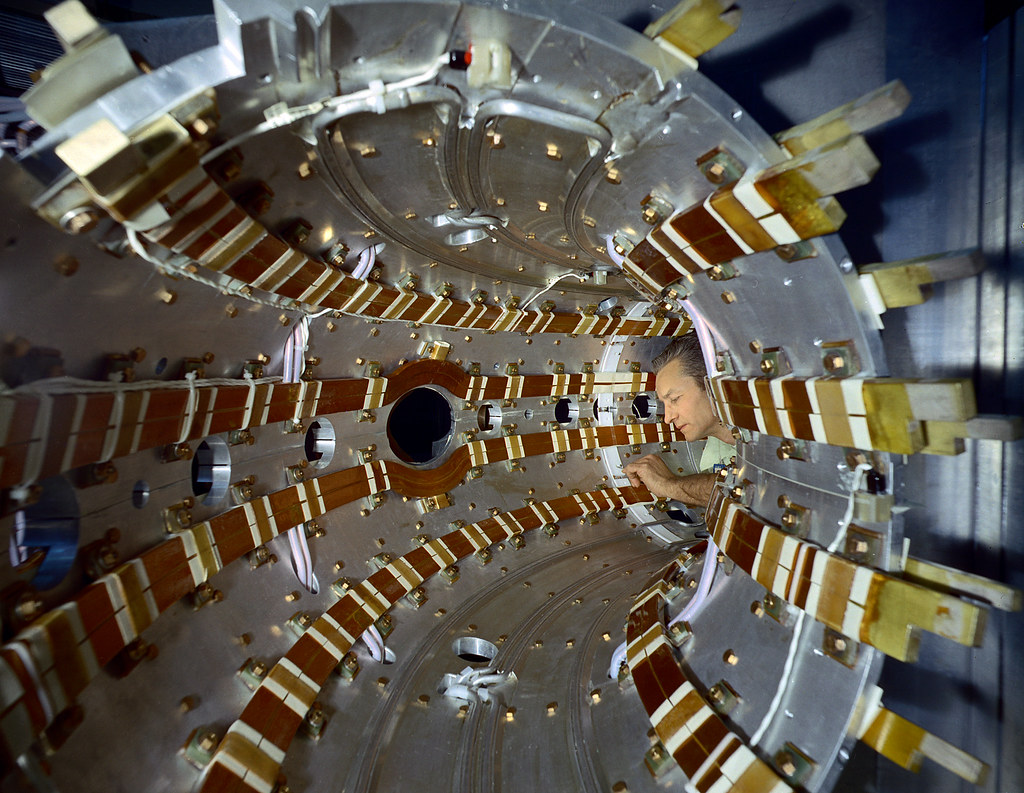
1973
ELMO Bumpy Torus
The original ELMO, which was either an acronym for "electron magnetic orbits," short for "electron mole," or possibly a reference to St. Elmo's fire, was a mirror machine that operated near the Physics Test Facility in 1961-62.
In the early 1970s, a novel approach was devised that combined mirror and toroidal concepts, where a number of simple magnetic mirrors, like the original ELMO, were arranged end-to-end in a circle, eliminating energy loss at the ends of the mirrors that could result in a stable toroidal plasma. Magnetic field lines were alternately squeezed together under the coils and expanded between them, creating a "bumpy" magnetic field shape, hence the name "bumpy torus."
The ELMO Bumpy Torus (EBT) began operating at ORNL in 1973, reusing four of the vacuum pumps from DCX-2. EBT served as the basis for work on electron cyclotron heating (ECH), a method of heating the electrons in a fusion plasma roughly analogous to heating food in a microwave.
The EBT device was upgraded to high-frequency ECH technology in 1979 and renamed EBT-S, for "scale-up." The concept was selected by the Department of Energy for further development as an alternative to standard mirror and toroidal devices, but improved diagnostics showed that it suffered from major losses of particles and energy, and would not be able to self-stabilize.
The EBT program was terminated in 1986, but yielded valuable fundamental information in several areas and provided the impetus and the opportunity for ORNL to become a leading institution in technology of high-power, high-frequency microwaves.
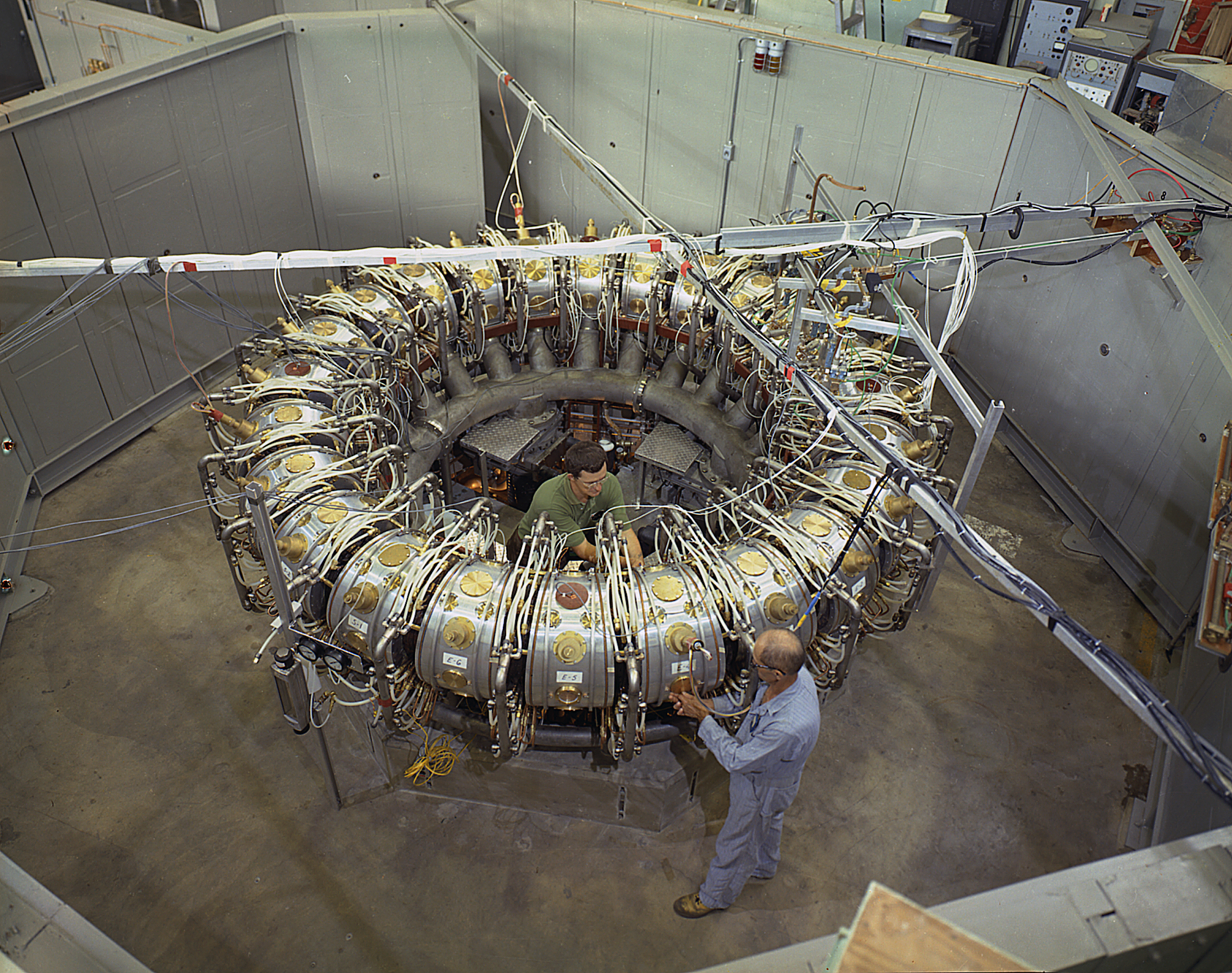
1974
Fusion & Computing Go National
Computing has been a part of ORNL's fusion work even before the lab had a computing facility of its own.
During the Project Sherwood era, group member Mozelle Rankin would frequently travel to Philadelphia to work on plasma physics problems on a UNIVAC machine. She would later travel to Argonne National Laboratory to work on the Oak Ridge Automatic Computer and Logical Engine, or ORACLE, before it was installed at ORNL in 1953.
Early minicomputers were used to process the data from experiments on DCX-2, and eventually data links were established between ORNL and Y-12, though they were often prone to fail during rainstorms.
Starting in the late 1960s, the division’s computing capabilities received a big boost. Over the next decade, computers were installed at ORMAK, EBT and the Large Coil Test Facility and networked to ORNL's Computer Sciences Division so data could be analyzed directly.
In the mid-1970s, future ORNL director Al Trivelpiece initiated a national computer network for fusion research, which launched in 1974 as the Controlled Thermonuclear Research Computer Center (CTRCC) at Lawrence Livermore National Laboraotry, with each major fusion lab installing powerful computers that both connected them to a nationwide network and provided excellent in-house computing resources. The CTRCC was eventually renamed to the National Energy Research Scientific Computing Center, operated by Lawrence Berkeley National Laboratory.
Today, high-performance computing is an essential part of ORNL's fusion research, with the lab's supercomputing resources helping to develop advanced modeling and simulation capabilities of next-generation fusion devices.
1976
Becoming the Fusion Energy Division
Following the debut of ORNL’s fusion efforts in Geneva, the former Project Sherwood group was officially named the ORNL Thermonuclear Project in 1959, then incorporated as the Thermonuclear Division in 1960.
Herman Postma, who started at ORNL as a student on the DCX project (as seen in the accompanying photo), became division director in 1967, then ORNL director in 1973.
As excitement and funding for fusion continued to grow, so did the division, as more test facilities and programs came to ORNL, and the lab continued to make significant contributions to magnetic fusion.
Eventually, the fusion program was moved from the facilities at Y-12 to the ORNL campus and was formally renamed to the Fusion Energy Division in 1976.
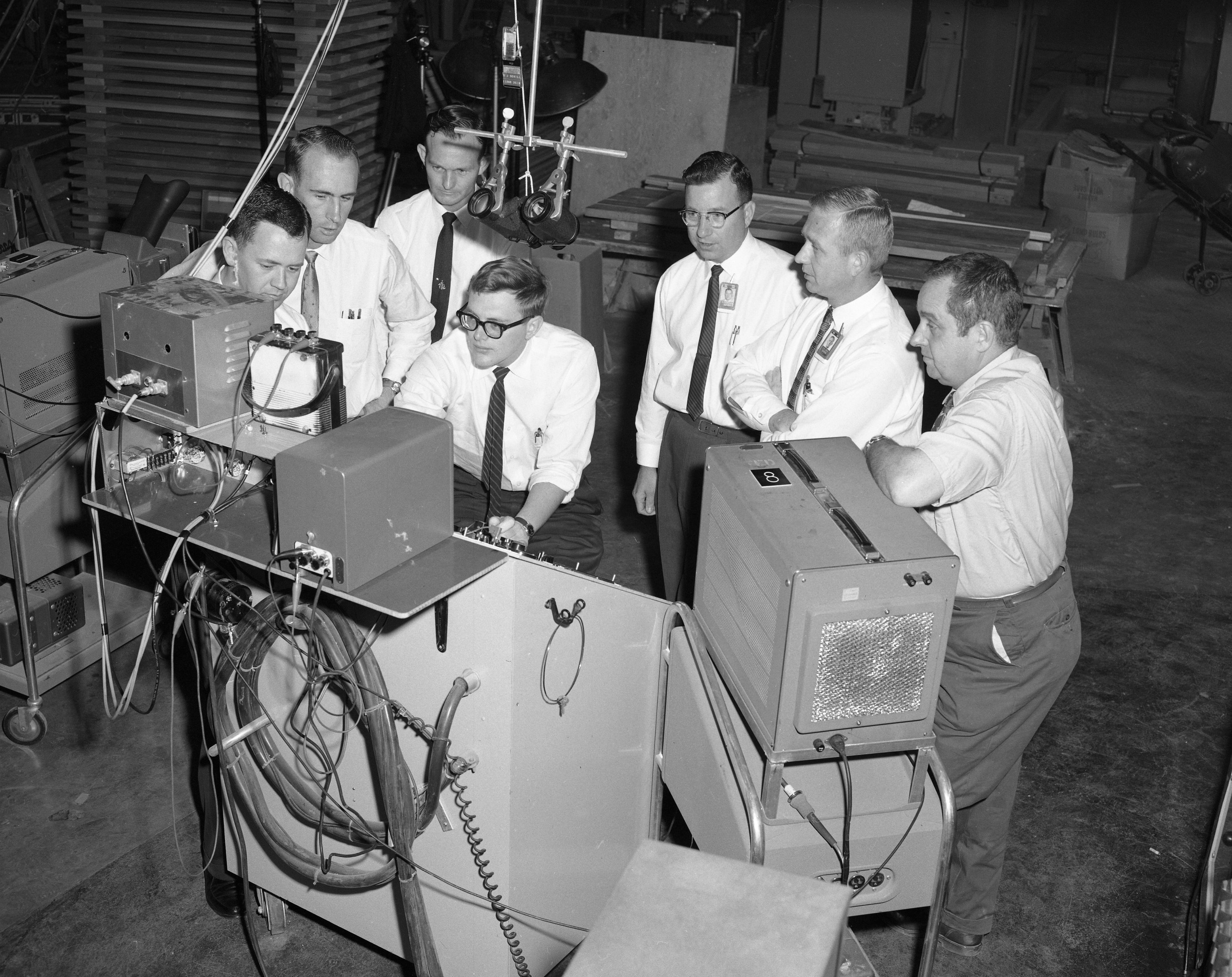
1976
ORNL pellet fueling bursts onto the scene
Pellet fueling – the technique of adding fuel to a fusion plasma via high-speed injection of frozen hydrogen pellets – was first pioneered at ORNL once it was made clear injecting molecular beams or gas would be insufficient to fuel a sustainable reaction.
The first experiments in pellet injection were performed on ORMAK in 1976 using miniscule spheres of frozen hydrogen isotopes injected into the vessel at 100 meters per second. These initial experiments led to new theories and further improvements, and it was determined that fuel pellets needed to be larger and faster.
To achieve the higher speeds, two new techniques were proposed by ORNL researchers: centrifuge acceleration and pneumatic acceleration. The first pellet extrusion and centrifuge system was built using a surplus turbo pump and Craftsman drill press, and successfully accelerated 150 pellets per second to velocities of 300 meters per second. The pneumatic injector used a narrow barrel with compressed helium gas to shoot the pellet also accelerated the pellet to 300 m/s, and was installed on the ISX-A tokamak in 1978, less than a week after it had been first demonstrated in the lab.
Both centrifuge and pneumatic injection were proven to be successful fueling methods, and ORNL's status as a leader in pellet injection technology was born.
1977
Impurity Study Experiment (ISX)
The Impurity Study Experiment program, which ran from 1977 to 1984, was a joint project with the General Atomic Company, now known as General Atomics.
The first device in the program, ISX-A, was inspired by ORMAK experiments in impurity control. Researchers designed ISX-A to determine the conditions at the edge of the plasma, study the effects of various materials on the edge plasma – and vice versa – and develop ways to control impurities and particles. The device was also used to investigate plasma shaping, ripple effects in magnetic fields, electron cyclotron heating, and new pellet fueling technology.
ISX-B, the successor, was among the first tokamaks to reach high beta. Beta is the ratio of outward pressure generate by the plasma to the inward pressure exerted by the magnetic fields and is an indicator of plasma performance efficiency. Experiments on ISX-B led to the observation that confinement becomes worse at high power and high beta. It operated until August 1984, with its lifespan extended a few extra months to help test beryllium components for the Joint European Torus (JET).
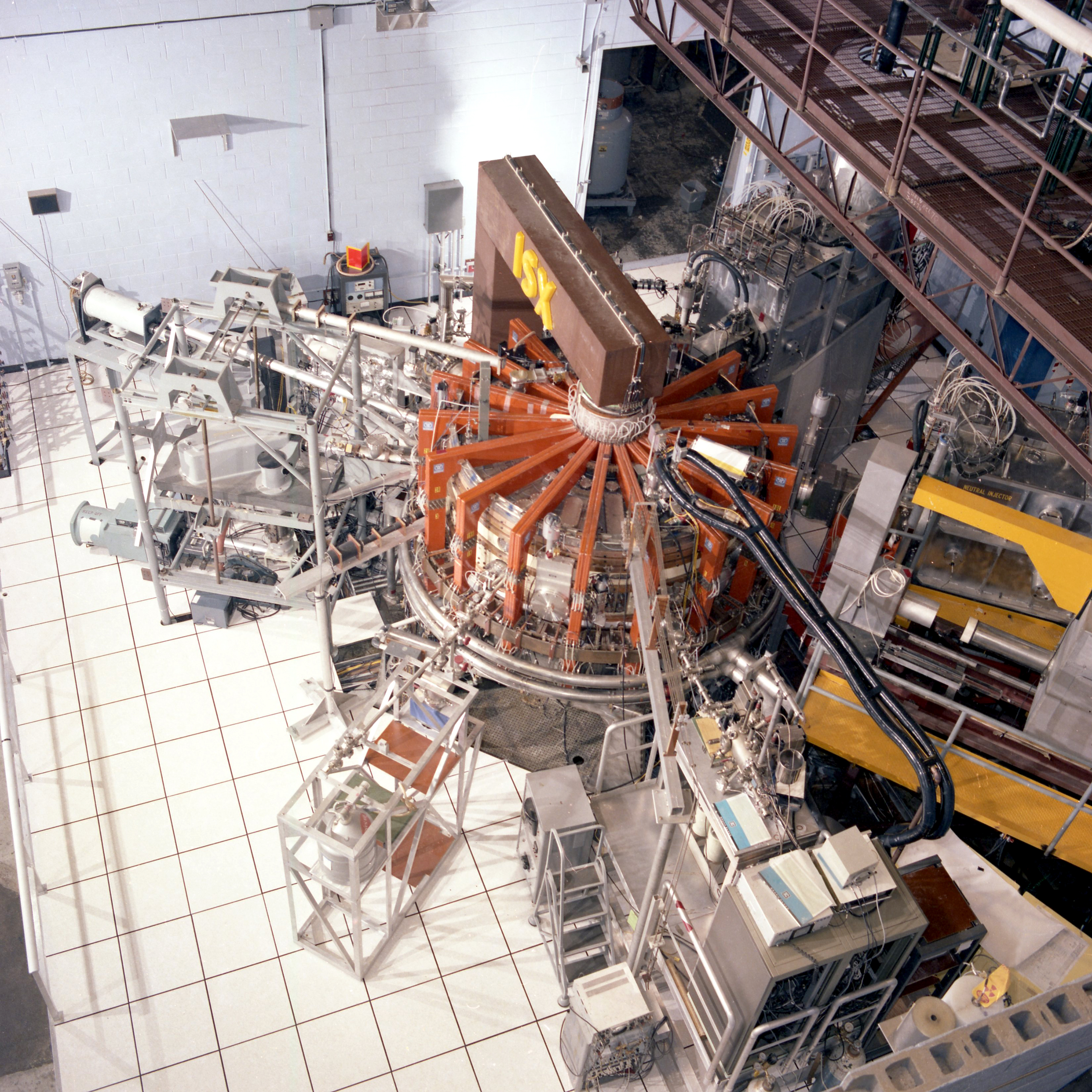
1977
Launch of the Large Coil Task
The need for strong magnetic fields to confine a fusion plasma had long been a vexing problem for fusion researchers, it would require magnetic fields 100,000 time stronger than the earth's magnetic fields, yet copper-based magnets could not achieve this without wasting a significant amount of energy. In 1972, a group of magnet specialists met at ORNL to estimate the time and cost to develop superconducting fusion magnets suitable for commercial fusion energy. A proposal was submitted to the Atomic Energy Commission to launch a superconducting magnet development program at ORNL, and in 1975, the Large Coil Program was initiated.
By 1977, the program had garnered interest internationally, and it was decided that several different magnet designs would be tested. The program was expanded into the international Large Coil Task, under the auspices of the International Energy Agency, with ORNL as operating agent. The facility under development would be called the International Fusion Superconducting Magnet Test Facility, also referred to as the Large Coil Test Facility.
The project would test six coils, three designed and built by U.S. manufacturers – Westinghouse, General Electric, and General Dynamics – and one each made by partners in Japan, Switzerland, and the European Community (EURATOM).
All of the coils were manufactured independently and successfully shipped to Oak Ridge for installation in the test stand in 1985. The Japanese coil even included a special "good luck charm" inside its crate that had been blessed at a Buddhist temple to ensure a safe journey as it was shipped via the Panama Canal to New Orleans and trucked to the lab.

1980
ORNL pellet fueling in big demand
The success of the pneumatic injector on the ISX-A device in 1978 attracted the attention of the international fusion community to ORNL's pellet fueling expertise. In addition to fusion experiments at ORNL, the pellet group began supplying injectors to other fusion laboratories, including the PDX tokamak at Princeton in 1980 and the Alcator C tokamak at MIT, which achieved a breakthrough in plasma density in 1983 using a pellet injector designed at ORNL.
Throughout the 1980s, new single-shot and repeating injector designs were developed for devices at Princeton, the Tore Supra superconducting tokamak in France, General Atomics' Doublet III device, now known as DIII-D, and the Joint European Torus (JET). Today, ORNL-designed pellet injection systems are found in more than a dozen major fusion facilities around the world, including ITER, KSTAR in South Korea, and the Wendelstein 7-X stellarator in Germany.
1985
Radiofrequency Test Facility (RFTF)
A radiofrequency (Rf) technology planning program began in 1981 at ORNL to define and carry out developments and facilities needed to support present and future fusion devices. A facility was designed to develop capabilities in the ion cyclotron range of frequencies, called ICRF, and ion cyclotron heating, which could heat the ions in a fusion plasma in a way that other heating methods, like electron cyclotron heating and neutral beam injection, could not.
The Radiofrequency Test Facility, which began operating in 1985, was meant to build on the expertise developed during experiments on the ELMO Bumpy Torus to help ORNL become a major player in ion cyclotron heating. RFTF was a large mirror machine that used two large superconducting coils and microwave sources repurposed from the EBT to generate a plasma, and a high-power Rf power source to conduct the ion cyclotron heating. RFTF eventually became a national facility for the testing and evaluation of steady-state, high-power ICRF systems and components.
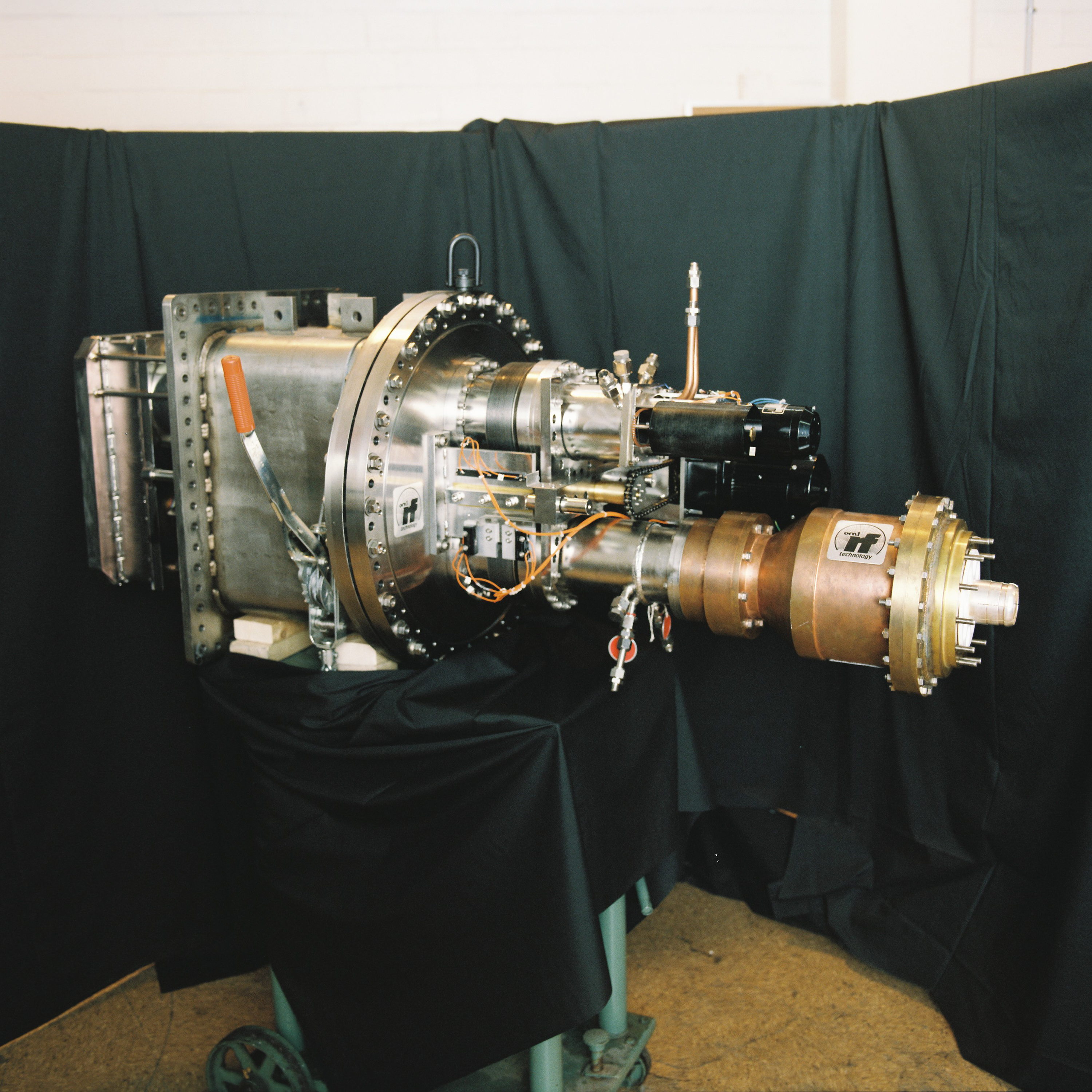
1987
Large Coil Task completed
The Large Coil Task was completed in September 1987, having successfully kept all six coils at cryogenic temperatures with liquid helium for 18 months. All of the coils exceeded their design goals, reaching magnetic fields of 9 tesla, about 180,000 times the strength of Earth's magnetic field, at full current.
The Large Coil Task was hailed both as a model of successful international collaboration and as a feat of engineering, with the Tennessee Society of Professional Engineers designating it as an outstanding engineering achievement in 1986.
The vacuum tank that contained the 400-ton array of coils was 35 feet in diameter and maintained a vacuum of one hundred millionth of atmospheric pressure, even with thousands of sensors and power lines breaching the sides of the vessel. It also had to withstand the tremendous forces generated by the magnets themselves, equivalent to the weight of a 100-car freight train. The refrigeration system supplied 250 liters of liquid helium per hour to the bath-cooled coils to compensate for boil-off, and 300 grams per second, about 35 gallons a minute, of high-pressure liquid helium to the forced-flow coils.
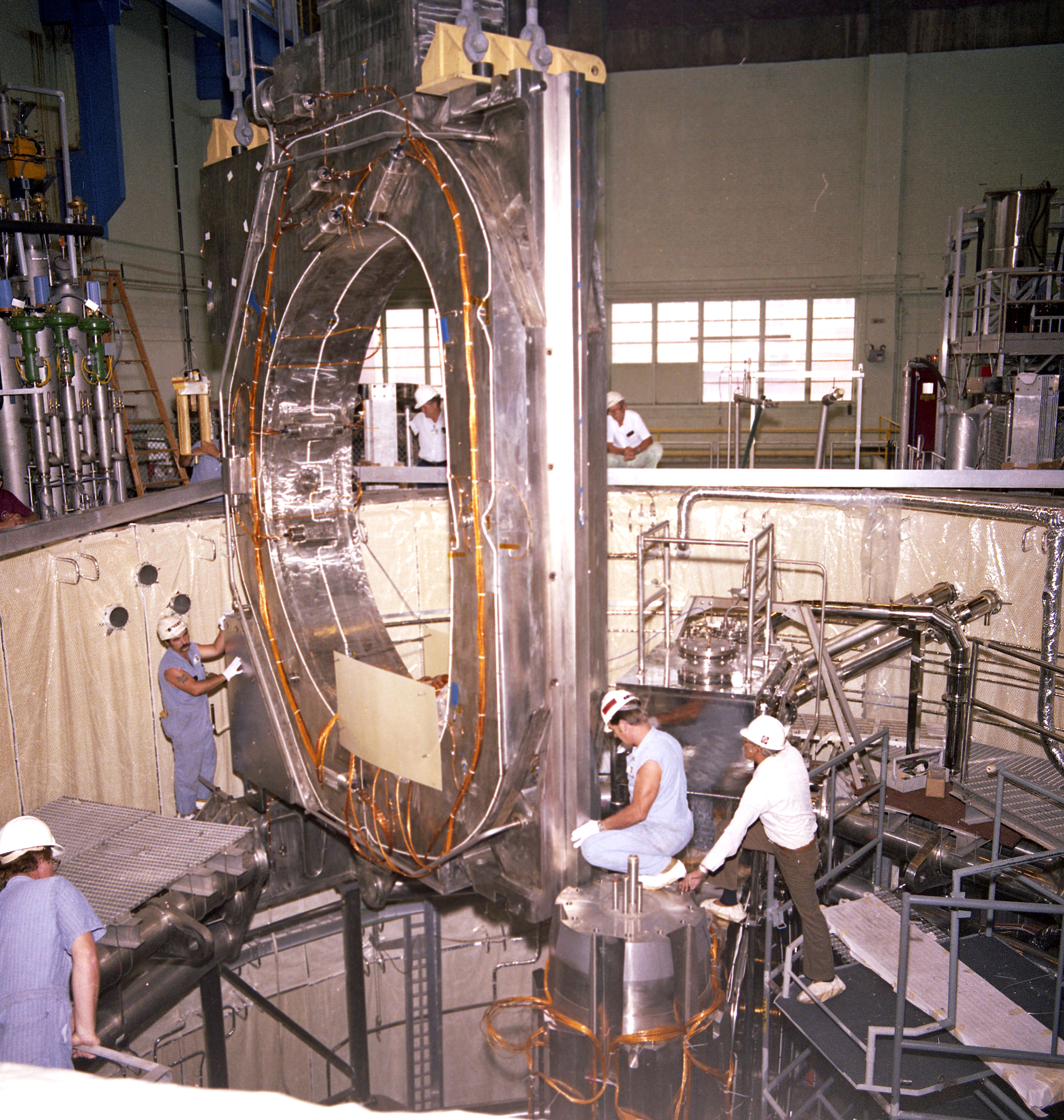
1987
ITER partnership formed
By 1985, the possibility of an expanded international fusion process as a form of science diplomacy was being explored through a number of summits between the U.S. and the U.S.S.R.
At the Reykjavik Summit in 1986, the Quadripartite Initiative Committee, comprised of the EURATOM countries, Japan, the U.S. and the U.S.S.R., was formed to move forward on this international collaboration, and in 1987, conceptual design studies formally began on the International Thermonuclear Experimental Reactor, now known as ITER.
The Fusion Engineering Design Center, established at ORNL in 1979 as a major engineering resource for the design of future fusion experiments, was tasked with leading many of the U.S. efforts, including configuration development, mechanical design of plasma-facing components and blankets, facilities, remote maintenance, heating and current drive design, and structural analysis.
Today, ITER is run by seven member parties, and the U.S. contributions to the project are managed through the US ITER Project Office in Oak Ridge.
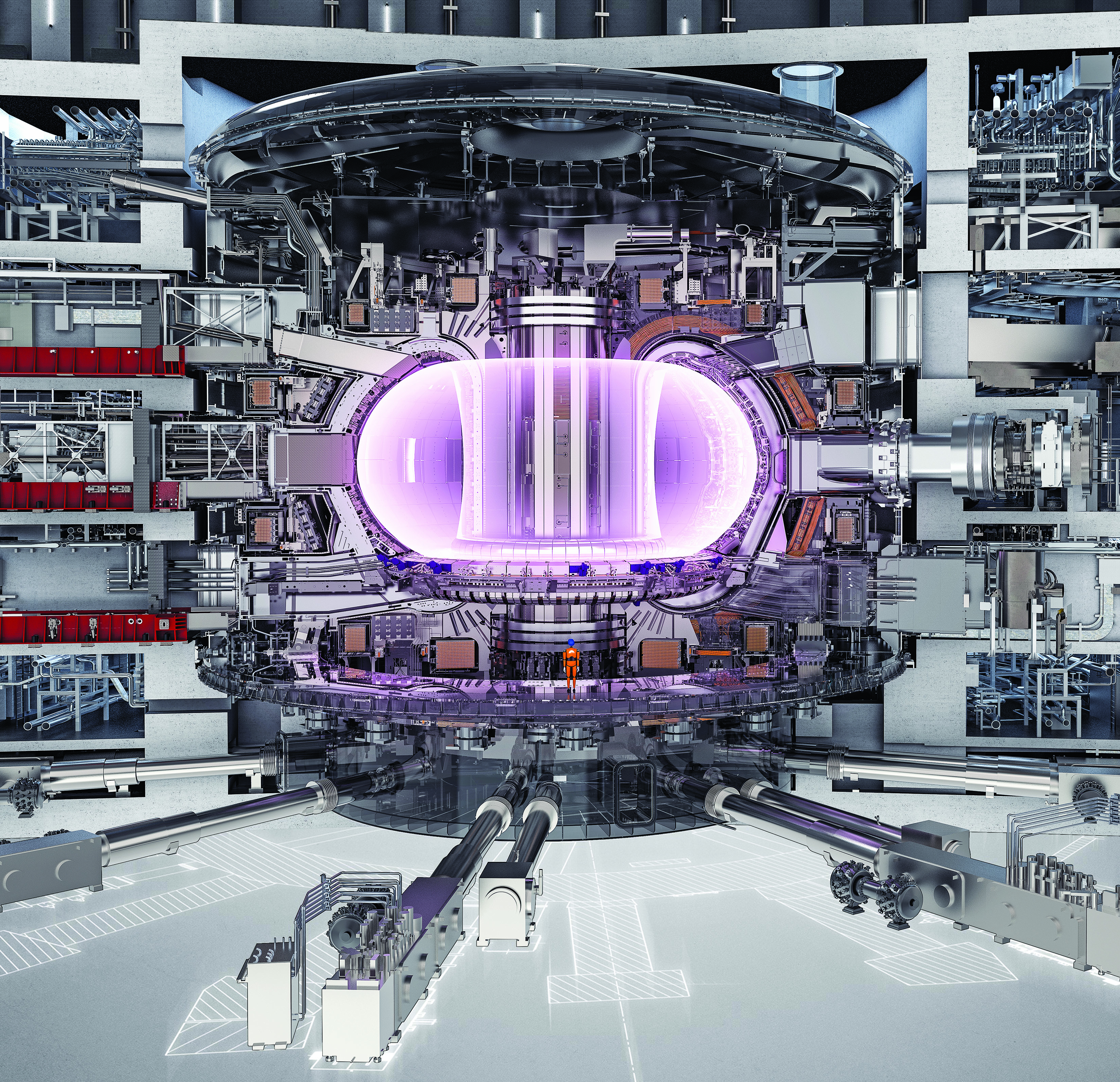
1988
Advanced Toroidal Facility
After the ISX program was shut down, the Department of Energy directed ORNL to investigate fusion device designs that were different from, and possibly better than, a tokamak., that could improve its beta ratio and efficiency and investigate steady-state operation.
The resulting design, the Advanced Toroidal Facility, was a type of stellarator known as a torsatron – picture a doughnut-shaped device, but twisted like a cruller pastry. Proposed and approved in 1982 and completed in 1988, the ATF was the world's largest stellarator when it began operation. It reutilized several components from the ISX-B device, including the power supplies, utility systems, diagnostics, and heating systems.
The goal of the ATF program was to better understand toroidal plasma physics and demonstrate the principles of high-beta, steady-state operation. It was designed to have a great deal of flexibility in the magnetic configurations that it could produce and could be used to study the effects of configuration properties on plasma performance.
1990
ORNL's Contribution to Plasma Physics
The theoretical understanding of the behavior of a fusion plasma has been an essential part of the fusion program since the beginning. A report on fusion energy research from the Office of Technology Assessment in the 1950s noted that "experimental results were often ambiguous or misinterpreted, and the theoretical understanding underlying the research was not well established."
ORNL's partnership between theory and experiment has its roots in the close collaboration of theorist Al Simon and experimentalist Rodger Neidigh in the early days of Project Sherwood. ORNL's theory program has led the development of transport theory for both stellarators and tokamaks, and made important contributions to the analysis and modeling of the Rf heating process, pellet fueling, magnetohydrodynamics, and current drive.
The basic collisional theory of neutral beam injection heating was developed at ORNL using results from ORMAK, and a number of stellarator modeling codes were developed for the ATF program that continue to be used to this day all around the world. ORNL also invented and developed a number of device designs, like the spherical tokamak and compact stellarator concepts, that were not built at the lab, but were further developed into experiments elsewhere, including the National Spherical Torus Experiment (NSTX) at Princeton and the Mega Ampere Spherical Tokamak (MAST) in the U.K.
1991
The end of the ATF program
The Advanced Toroidal Facility program operated until November 1991. From the start, it yielded valuable insights into plasma physics and currents, both intentional and not.
When the ATF first came online, there was a double dose of bad news-good news. The bad news was that a design oversight caused an error in the magnetic fields. This actually turned out to be good news and an opportunity, however, as the error had caused the ATF to immediately operate in a "second stability regime," which only been predicted theoretically to that point. This second stability regime could only be achieved with a high beta ratio and strong magnetic fields, as there was a "sea" of instabilities between low and high beta ratios.
While the ATF was designed to test the existence of this regime, it was not expected to achieve it so soon. This serendipitous achievement provided experimentalists and theoreticians a rare chance to identify and characterize the second stability regime.
The ATF also led to several important studies in plasma currents and configurations, and facilitated national and international collaborations on new diagnostic developments.
2014
Proto-MPEX
Materials inside a fusion reactor, especially those that will be close to or facing the plasma, must withstand extreme conditions, including high temperatures, strong magnetic fields, and bombardment by high-energy neutrons. The plasma itself can fundamentally alter the structure of a material, and the material can generate byproducts from this interaction that affect plasma performance.
To study these effects and find suitable material candidates, fusion scientists at ORNL established the Material Plasma Exposure Experiment, or MPEX. The forerunner to the main MPEX device, known as Proto-MPEX, operated on the Fusion Energy Campus from 2014 to 2021. Like many of ORNL's other fusion devices, Proto-MPEX repurposed components from past experiments, including the ELMO Bumpy Torus and the Advanced Toroidal Facility.
While the main MPEX device will run in steady-state operation, Proto-MPEX ran only in short, intense bursts, executing almost 30,000 plasma pulses in its lifetime. One of Proto-MPEX's key accomplishments was the testing of electron Bernstein wave heating (EBW), a novel heating concept that uses a microwave source to heat high-density magnetized plasmas. It also successfully demonstrated ion heating in a high-density linear plasma for the first time.
2021
MPEX fabrication begins, fusion materials at the forefront
With the MPEX design approved and the project greenlit by DOE, ORNL began the process of procuring and fabricating some of the major long-lead components and systems for the device, including the superconducting magnet system, gyrotron microwave sources, and high voltage power supply.
While MPEX represents the future of fusion material research, ORNL has a long history of research and development of advanced fusion materials dating back to the Fusion Reactor Technology Program in the mid-1970s. The program included studies of materials compatibility, mechanical properties, and radiation effects of plasma-facing components, studies of neutron radiation damage and neutronics calculations, and investigations of tritium behavior and processing techniques.
Today, the fusion materials program at ORNL brings together researchers from fusion energy, materials, neutron science, and isotope technology to develop new alloys and composites for components like the first wall, blanket and divertor. Researchers are able to simulate the neutron damage from a fusion reactor by irradiating materials in the High Flux Isotope Reactor and examine the material properties with a suite of advanced microscopy and material characterization tools. Materials scientists also conduct corrosion studies of coolant and breeder materials in the blanket to find the best balance of performance and reliability.
2025
MPEX continues assembly
As of mid-2025, the Material Plasma Exposure eXperiment is completing procurement and device assembly is underway, with systems like the high voltage power supply installed and accepted, and a projected completion date of January 2028.
Once complete, MPEX will be a world-leading resource that will enable fusion researchers to answer critical questions in the field of plasma-material interactions and develop robust plasma-facing materials and components.
MPEX will combine electron and ion cyclotron heating systems to produce linear plasmas up to 150,000 Kelvin and magnetic fields of 2.5 tesla, much like a steady-state fusion reactor, and will have a suite of instrumentation nearby for in-house analysis of exposed materials. It will be capable of replicating the effects of months or years inside a fusion reactor in just a few weeks, letting researchers model a component's entire lifespan in a condensed timeframe and rapidly iterate on new materials.
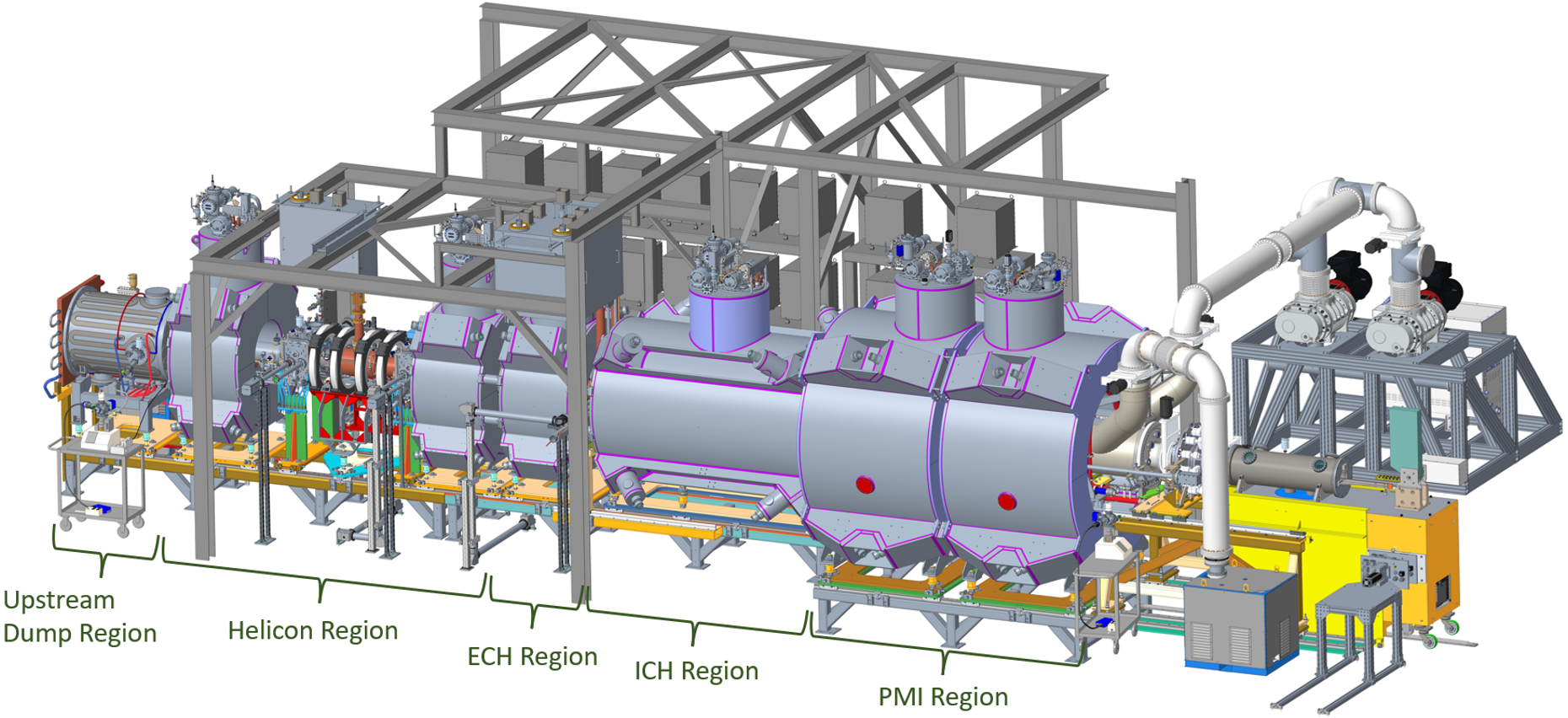