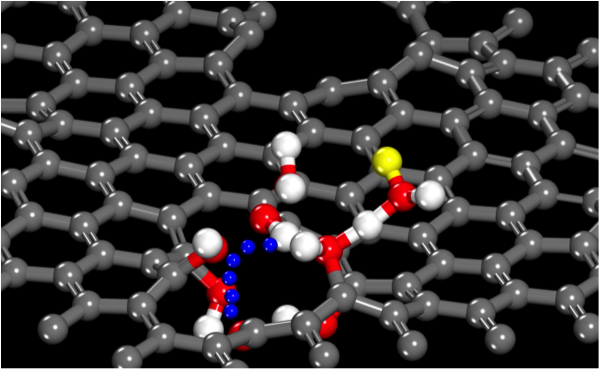
But now, a FIRST EFRC team combined the latest synthesis, computational, microscopy, and laser methods to show that certain types of atomic scale defects in the material enable the selective transfer of protons, which follow a “bucket-line” transfer mechanism, hoping from a water molecule to hydroxyl (OH) groups terminating the broken carbon bonds surrounding the defect, to water on the other side of the membrane, driven by a difference in pH (-log[H ]) across the membrane. The protons can migrate through an array of water molecules and OH groups by the sequential making/breaking of hydrogen bonds, such that the oxygen atoms of the water and OH groups do not have to physically move in order to enable diffusional proton transfer. This is the classic “Grotthuss mechanism” proposed in 1806 by Theodor Grotthuss, even before the atomic/molecular structure of water was understood.
Experimental observation of the unimpeded passage of protons through the graphene was achieved by using a nonlinear laser optical method known as Second Harmonic Generation (SHG) to monitor the change in surface charge of a fused silica substrate wetted by a nanoscale film of water, that was then capped by a single graphene layer, with this multilayer interface then immersed in bulk water. Change in pH of the nanoscale water film causes change in the silica surface charge, which results in changes in the SHG signal. We discovered that the response of the interface to changes in pH in the bulk water ABOVE the graphene film was very fast, indicating that the graphene film did not impede the transfer of protons to the nanoscale water layer between the graphene and the silica surface.
High resolution Scanning Transmission Electron Microscopy (STEM) was used to carefully characterize the large, single graphene layer transferred to the silica surface, and this demonstrated that there were not nearly enough ‘macroscopic’ holes in the graphene to allow such rapid and uniform pH equilibration across the membrane. However, STEM also identified a rare by fairly uniform distribution of atomic scale defects, involving just a few missing carbon atoms. Extensive computational calculations and simulations at the quantum level (DFT) and classical reactive force field level (ReaxFF) demonstrated that on OH-terminated defects were able to pass protons through the membrane via the Grotthuss mechanism with a sufficiently low energy barrier to allow efficient transport at room temperature. ReaxFF simulations also demonstrated that there were enough of this type of defect to permit rapid equilibration of the pH of the nanoscale water film with the bulk water phase above the graphene membrane. These computational approaches were also used to demonstrate that even the smallest molecular species, helium (He) and hydrogen (H2) would encounter much higher energy barriers, effectively preventing their passage through such defects, except at exceedingly high pressures. Thus, the OH-terminated 4-carbon defects identified in this study selectively allow only proton (aqueous H ) transfer across the single layer graphene membrane.
This discovery (of the world's thinnest proton channel) opens up the possibility for using graphene containing such tiny defects in next-generation fuel cells, which currently rely on proton transfer across polymeric membranes hundreds of microns thick, that also undesirably allow transport of water or alcohol, which reduces the efficiency of hydrogen or methanol fuel cell operation.
The upshot is that perfection is not needed when it comes to the synthesis of proton-selective graphene membranes, hopefully enabling the manufacturing of less expensive fuel cells.
Contact: David J. Wesolowski, wesolowskid@ornl.gov
Jennifer L. Achtyl, Raymond R. Unocic, Lijun Xu, Yu Cai, Muralikrishna Raju, Weiwei Zhang,
Robert L. Sacci, Ivan V. Vlassiouk, Pasquale F. Fulvio, Panchapakesan Ganesh, David J. Wesolowski, Sheng Dai, Adri C.T. van Duin, Matthew Neurock & Franz M. Geiger, Nature Comm., 2015, DOI:[10.1038/ncomms7539].