The human element: Expanding the periodic table
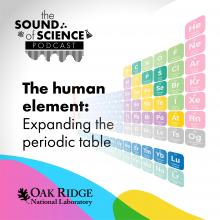
It’s the International Year of the Periodic Table. Did you know Oak Ridge National Laboratory has been helping shape the periodic table for more than seven decades? The lab has discovered three elements, and contributed to the discovery of several more through its isotope production and detector technology. In this episode, you’ll hear from a some of the team that helped discover one of the table’s newest elements, tennessine.
Transcript
[MUSIC/INTRO]
JIM ROBERTO: This is big science.
ROSE BOLL: Who would put on their bucket list that I’m going to be part of a discovery team for an element?
CLARICE PHELPS: It’s what research, what science is all about, solving problems.
JIM ROBERTO: This story is filled with intrigue.
[THEME MUSIC]
JENNY: Hello everyone and welcome to the Sound of Science, the podcast highlighting the voices behind the breakthroughs at Oak Ridge National Laboratory.
MORGAN/JENNY: We’re your hosts, Morgan McCorkle and Jenny Woodbery.
[MUSIC]
JENNY: It’s the International Year of the Periodic Table. For 150 years, the periodic table has been a guiding force for chemists around the world.
MORGAN: As you may remember from your high school chemistry class, Dmitri Mendeleev developed the periodic table to organize the elements and give us an understanding about their properties and chemical interactions.
JENNY: And just in case you don’t remember your high school chemistry class, elements are defined by the number of protons in their nucleus. This is also known as their atomic number; hydrogen has one proton, helium has two and so on.
JENNY: When it was first published, the periodic table had around 60 known elements. Today there are 118, and scientists are constantly pushing the boundaries of chemistry and physics to add to that number.
MORGAN: Discovering a new element is no easy feat. It can take years – even decades – of research and experimentation to achieve. And many who try, never succeed.
JIM ROBERTO: Any element on the periodic table, it just appears to be a substance and its position helps you describe what its properties might be. But there's actually a story behind that. Because for virtually all of the elements there have been stories of heroic struggles, competition between groups, unfortunate stories where people actually fabricated results and just stories of individual effort.
JENNY: That’s Jim Roberto. He’s a recently retired ORNL associate laboratory director who played a role in the discovery of one of the table’s newest elements, tennessine.
MORGAN: Since the beginning, Oak Ridge and its unique research facilities have been supporting the quest for new element discovery.
JENNY: In fact, the lab has been credited with three element discoveries, and, by supplying isotopes and detector technology, it has assisted with several more.
MORGAN: We wanted to explore some of the stories behind the elements ORNL has played a role in discovering – the first being promethium, or element 61, in 1945.
ROBERTO: The role of ORNL in the periodic table goes all the way back to the Graphite Reactor in the early days. And the Graphite Reactor, the first continuously operated reactor in the world, as part of the nuclear reaction process created fission products. Very innovative scientists at that time understood that those fission products were in about the same atomic number range as a missing element in the periodic table.
Now we think of the known elements that we deal of every day in the periodic table as they've always been there. But in fact, several of the isotopes in the known periodic table, before we get to these more exotic ones, are radioactive. And so there were gaps. And one of the gaps was at element 61.
JENNY: Lab chemists were able to identify element 61 from the fission products of the Graphite Reactor because they knew where it should fit on the periodic table and they knew what its properties should be.
ROBERTO: They were able to convince the scientific community that this was the missing element 61, and as a result of that they got the honor of naming it. They named it promethium because it was born of fire, born of the nuclear fire in the reactor. And that is the first recognition of ORNL in the periodic table.
JENNY: That recognition didn’t arrive until 1947 because of the secrecy required by the Manhattan Project – similar to the elements, plutonium, americium and curium discovered during the war by renowned chemist Glenn Seaborg and his colleagues at University of California at Berkeley. After the war, the research community began looking at ways to study and produce even heavier elements and isotopes.
MORGAN: Seaborg, who by the 1950s had earned a Nobel Prize and was chairman of the Atomic Energy Commission, presented a vision for a reactor with a very high neutron flux – or intense flow of neutrons. He lobbied for the reactor to be built at Oak Ridge. This led to the construction of the High Flux Isotope Reactor, or HFIR, in 1965.
JENNY: When HFIR was built, it had the highest thermal neutron flux of any reactor in the world. This enabled it to produce isotopes like californium that are very difficult to make. Next door, the Radiochemical Engineering Development Center, or REDC, had a unique capability to perform high precision separations to get very pure products of these isotopes.
MORGAN: So why were HFIR and REDC so important for element research?
ROBERTO: In the early days after the 1940s of producing new elements, they were produced by irradiating targets with neutrons or with protons or with deuterons to add a few protons to an existing element and build the next one.
JENNY: The basic idea is that researchers were making new elements by smashing a beam of one element into a so-called target made of a different element. If the conditions were just right, the two would combine to create a new, heavier element.
MORGAN: This sounds simple but it only got more challenging as they went up higher on the periodic table. As researchers started to make heavier elements, they needed heavier targets. That led them to start using heavy isotopes such as the ones that could be produced at HFIR, like californium.
JENNY: Fast forward 50 years later, HFIR continues to produce the highest neutron flux in the world and is still being used for new element discovery.
CHRIS BRYAN: I’m Chris Bryan, the irradiations manager or experiments manager at the High Flux Isotope Reactor. So, in order to create all these new elements you need to have a place where you can get a lot of neutrons delivered to targets in a fairly short amount of time, and the unique thing that HFIR brings is a very high neutron flux. So, for many of the element discoveries that involve bombardment, the target materials had been fairly easy to produce and could be produced at other reactors. For these elements that are up here in the 117 range and higher, the target materials become more difficult to produce. Those target materials have to be produced at reactors that have this very high neutron flux. And with really only two reactors in the world – the Russian SM3 reactor and HFIR, there aren’t a lot of places to choose from to make these target materials.
JENNY: HFIR and neighboring REDC work in tandem to produce radioisotopes. This process begins with targets that are produced at REDC. Targets are aluminum tubes that hold the material that will be irradiated in the reactor. Operators use special tools to place the targets into the reactor – which is located about 20 feet under a pool of water.
BRYAN: It’s kind of like laparoscopic surgery inside a reactor building. You’re dealing with 20-foot tools and trying to manipulate very small capsules at the end of them. Irradiating the capsules is a routine thing that we do all the time.
JENNY: This process may be routine, but the nuclear physics that takes place inside the reactor is pretty unique. We talked to Julie Ezold, who manages the californium program at ORNL, to break down what just happens inside these capsules.
JULIE EZOLD: When the neutrons are interacting with the material inside those capsules, you have about four options of the neutron interacting. It can come in and it won’t interact at all, it will hit that atom and just bounce off and that’s due to the different energies. Or depending on the characteristics of your material inside the capsules, the neutron can be captured. Now in capturing we have three options. One, you split apart. You fission. And now that element is not that element anymore – it’s broken into different elements. It can capture and give off the extra energy as a gamma, and then convert into a new isotope. So same number of protons, now we got one more neutron.
MORGAN: And there’s one more option that’s truly crazy. The neutron is captured with so much extra energy that it actually converts into a proton. The extra energy is given off as a beta particle, essentially an electron, and you’ve moved one element up on the periodic table.
EZOLD: So, in essence, beta decay is an alchemist’s dream in that it’s changing from one element to a different element. We’re not turning lead into gold, but I can convert curium to berkelium, then berkelium to californium, and californium to einsteinium, etc.
JENNY: After the material come out of the reactor, it makes a 700-foot journey to the hot cells at REDC. It is loaded into a 25-ton shipping container called the “cue ball.” Why? Because it’s white and round and literally looks like a cue-ball from the game of pool. The container is then placed on a tractor trailer and driven to the neighboring facility.
MORGAN: Wondering what a hot cell is? The hot cells are shielded workspaces that allow technicians to safely work with radioactive material.
JENNY: Technicians are separated from the material through four feet of leaded glass that’s filled with oil. They use mechanical arms to handle and manipulate the radioactive material. So, it’s kind of like playing one of those claw games at an arcade. Clarice Phelps is a researcher at REDC and is involved in processing the radioisotopes produced at HFIR.
CLARICE PHELPS: We are currently in the control room of Building 7920, which is the Radiochemical Engineering Development Center. Our hot cells are able and capable of handling high levels of radioactivity processing, and these buildings have been around for over 50 years, and so that is a testament to how back when they were building this facility, the forethought they put into what this building could be capable of.
MORGAN: In the early 2000s, the unique capabilities of HFIR and REDC drew the attention of Russian researcher Yuri Oganessian, who had pioneered a revolutionary technique called “hot fusion.”
JENNY: But let’s back up for minute. In the late 1990s, element discoverers were at an impasse. They had made their way up to element 112, but the techniques to create new superheavy elements were reaching their limits. It was getting harder and harder – and longer, to the point of a single experiment lasting years to get even a few atoms of a new element.
ROBERTO: So this was what many thought was the end of the field. No more elements were going to be discovered because you could not run those experiments that long.
MORGAN: Oganessian, a researcher at the Joint Institute for Nuclear Research in Dubna, Russia, developed a risky approach that had been theorized but never attempted. His idea was to smash a neutron-rich calcium beam into an actinide target – actinides are heavy radioactive elements.
JENNY: In theory, this would create a compound nucleus of a new superheavy element. The risk was that compound nuclei tend to immediately break apart by fission, meaning you might lose the chance to actually create a new element.
ROBERTO: And so this is called the hot fusion technique. Its use was pioneered in Russia in a great scientific gamble. Everyone else was doing different things. There was lots of skepticism, as there often is when you change directions in science.
JENNY: Oganessian proved the success of hot fusion with the discovery of element 114 in 1998. In the early 2000s, he had his eye on trying it out in the hunt for element 117. But first he needed berkelium-249.
MORGAN: Berkelium-249 is an exclusively man-made material that is a byproduct of californium-252, another synthetic radioisotope used in medicine and industry. HFIR is one of the only places in the world you can produce californium.
JENNY: Why berkelium? It boils down to basic math. Berkelium has an atomic number of 97, and Oganessian had plans to smash the berkelium with a beam of calcium ions produced in an accelerator facility in Russia. Calcium has 20 protons in its nucleus. So add them together and, in theory at least, you should get element 117. So, he proposed a partnership with ORNL to look for the new element.
MORGAN: The lab started the campaign to produce berkelium-249 for the experiment in 2008.
PHELPS: It actually starts years beforehand with preparing curium targets to be irradiated, and so the curium gets irradiated at HFIR, produces californium, and there's still come curium in there, and there's also some berkelium, some einsteinium and some fermium. And so we do a separation to separate those radioisotopes – from each other, and then the berkelium fraction taken from those irradiated targets is then processed in the glovebox like we did for super-heavy element program and for tennessine.
MORGAN: The californium targets are irradiated in HFIR for several months and then transferred over to REDC for the painstaking separations and purification process. Rose Boll is a radiochemist at REDC who helped purify berkelium in multiple campaigns.
ROSE BOLL: You have to be a little bit OCD in this job 'cause you've gotta be very detail-oriented, you're working in a confined space within a glovebox, you only have so much working area. You have to keep it ultra-clean if you wanna come up with ultra-clean material when you come outta that glovebox. So – and you've gotta think through each of the steps. You don't wanna take in more material than you need. It occupies your space. It then becomes waste that you have to have a way out for. So, it's a lotta planning, a lot of technique, the technique, so I think that all contributed.
JENNY: Next, they needed to send the material to Russia for the element 117 experiment. But here’s the thing with radioisotopes like berkelium-249, they start decaying right away. So, you’re basically in a race against the clock from the moment the material comes out of the reactor.
ROBERTO: It takes about six months to go through the entire process of letting the targets cool down and doing the separations and shipping them to Russia. A significant amount of the material had already been lost and we knew the experiment would take at least six months. So, it was very important to do this as quickly as we could.
MORGAN: With researchers already racing against the clock, an unexpected shipping gaffe didn’t help matters. Somehow the material was mislabeled during the shipping process and ended up taking three flights over the Atlantic before arriving at Joint Institute for Nuclear Research in Dubna, Russia.
JENNY: Despite the delays, the experiment for element 117 began in July 2009.
ROBERTO: It ran for about six months and about six atoms of tennessine were seen. At the same time, tennessine decays into element 115, which was also a new element. And so the discovery actually resulted in two new elements. So, if you bombard a target of berkelium with calcium 48 ions at very high intensities for six months and only see six atoms, you're actually seeing the product of trillions of interactions resulting only in the production of six atoms.
JENNY: With only six atoms produced – how do you know you’ve actually found a new element?
MORGAN: Well that’s where the lab’s expertise in detector technology comes in to play. ORNL has been engaged in state-of-the-art detector technology since the 1940s. In the area of new element discovery in the 1970s, ORNL nuclear physics detection capabilities were used to help confirm elements 102, 103, 104, and 105.
JENNY: In the era of superheavy elements, the lab’s digital detection technology is very important in terms of being able to accurately monitor these fast-decaying products from these reactions.
MORGAN: We talked to a nuclear physicist who helped develop these sophisticated detectors.
KRZYSZTOF RYKACZEWSKI: My name is Krzysztof Rykaczewski and I think last time I checked I was distinguished senior researcher or something like that. Comes with age you know.
We developed a very unique system here for these very difficult studies when the nuclei were living even at the level of a microsecond. In this first experiment we got six events during 150 days, so it’s a rare process. So you better be prepared if the event comes, you detect it. And you detect it in a way that it will be unambiguous to say, oh look there is absolutely new evidence, and this is our new element.
JENNY: To make sure this happened, Krzysztof and his team developed new detectors for the experiment. Then they layered four to five detectors on top of each other so there would be no chance they’d miss detecting the new element. And they didn’t.
RYKACZEWSKI: And so far every superheavy event we observed was confirmed by independent analysis of two groups.
MORGAN: The team had unequivocal proof of element 117 in 2010. However, as Jim Roberto explains, it would take another six years for the discovery to be officially recognized and placed on the periodic table.
ROBERTO: The acceptance of an element to go on the periodic table is done by a committee of the International Union of Pure and Applied Chemistry and the International Union of Pure and Applied Physics. And they quite appropriately are very rigorous stewards of the periodic table, and they looked at our discovery in 2010, when the publication came out, and it wasn't until 2016 that they actually approved these elements.
JENNY: When it came time to name the new element, tennessine was chosen as a nod to the significant contributions of ORNL and its partners in the state of Tennessee.
ROBERTO: An interesting side bar of that is why is it tennessine i-n-e and not tennesium, i-u-m? This actually relates to chemistry and if you look at the periodic table, element 117 fits in that column where chlorine and fluorine and others are. And it is the preference in chemistry that all elements that fit in that column should end in i-n-e. And so that's why it's tennessine.
MORGAN: Element 117 may be called tennessine, but its discovery wouldn’t have been possible without international collaboration. Jim says that’s one of the most rewarding aspects of this kind of work.
ROBERTO: Element 117 could not have been discovered by the Russians on their own because they did not have access to the target material. And element 117 could not have been discovered in America alone because we did not have the right type of accelerators for doing this experiment. But by working together we were able to do it.
JENNY: After seven decades of shaping the periodic table, ORNL researchers aren’t resting on their laurels. They’re already involved in experiments to look for elements 119 and 120, using targets produced at ORNL and irradiated in accelerators in Japan and Russia.
ROBERTO: Those experiments are ongoing now. There appears to be a path to get to element 119 and element 120, although these experiments are likely to take a year. Beyond 120 it becomes more complicated and we're going to have to find another breakthrough, because we don't think the periodic table ends at 120.
MORGAN: Discovering a new element is huge deal, and we wanted to know what it feels like to make such a lasting contribution to science.
BOLL: You know, who would put on their bucket list I'm gonna be part of a discovery team for an element? I mean, I would never have thought that would happen in my lifetime. And my role was to purify the berkelium which became the target for the production of the tennessine. And I was doing my job.
BRYAN: It’s not something you ever think you’ll do in your lifetime. It’s just fascinating and exciting. I think the whole experience is humbling, because despite the fact we get great credit for being part of the team that discovered this element, there are hundreds of people behind the scenes that make the reactor operate, that make the hot cells operate, that make this whole machine function to be able to produce a target material like this.
ROBERTO: In many respects if you go into science, it's nice to dream about this but it can't be an expectation. There is both hard work and luck involved and being in the right place at the right time. But given all of that it's a lot of fun to discover a new element.
[MUSIC]
JENNY: Thank you so much for listening to this episode of the “Sound of Science.” We’d love to hear your feedback. So leave us a comment or review.
MORGAN: And don’t forget to subscribe so you get the latest episodes when they’re released.
JENNY: You can find us on Apple podcasts, Google Play Music or Spotify. And if you want even more up-to-them minute news from the lab, follow ORNL on Facebook, Twitter, Instagram and LinkedIn.
MORGAN: Hopefully it won’t be too long until we’re announcing the discovery of element 119.
JENNY: Until next time!
[MUSIC OUTRO]