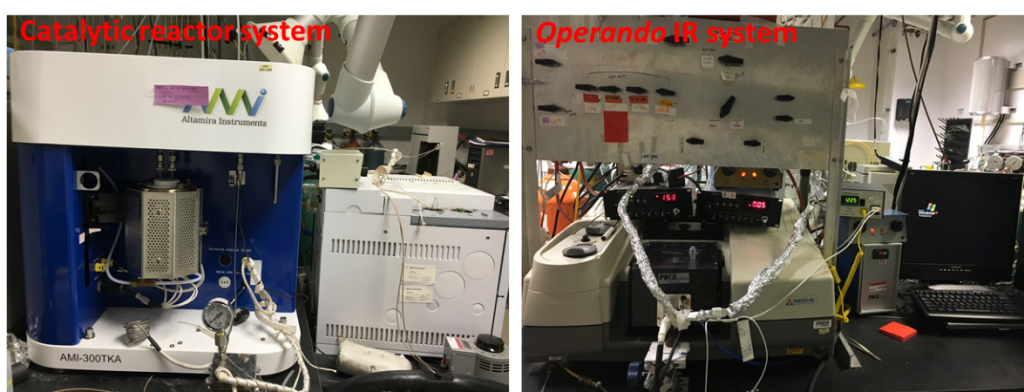
Researchers use catalytic reactors to evaluate the catalytic performances including conversion, selectivity and stability of various heterogeneous catalysts. Infrared spectroscopy is powerful in providing information on the surface reaction intermediate and the chemical nature of catalytic sites.
Science Overview
The catalytic reactor systems can evaluate the performances of catalysts in both steady state and temperature-programmed (TP) modes. The steady state tests including transient kinetic analysis via isotope labeling provide intrinsic catalytic behaviors in rate, selectivity, and stability while the TP measurements gives insights on the evolution of catalysts during reduction, oxidation and other conditions, the adsorption and reaction of reactants/products and the dispersion of oxide and metal sites.
The operando IR spectroscopy can probe surface adsorbates and chemistry (via IR) under actual working conditions while simultaneously determine catalytic activity and selectivity (via online mass spectrometer).
Applications
The catalytic reactor systems have been used to evaluate various heterogeneous catalysts in terms of their performance in conversion, selectivity and stability in various gas phase reactions. These catalytic systems can also be investigated mechanistically via the operando IR system. Previous work includes studying thermal catalysis over transition metal oxides, zeolites, carbon nanostructures, and supported metals.
Specifications
- AMI-200 and AMI-300 (with SSITKA capability) coupled with online GC and MS. Temperature and pressure ranges: 173 – 1200 K, up to 1 atm
- IR system: coupling with online MS, diffuse reflectance reactors handling temperature 120 – 1000 K and pressure up to 1 atm.
- Gas flow: various oxidative, reductive and reaction feeds
Recent Publications
Liu, X.; Hood, Z. D.; Zheng, Q.; Jin, T.; Foo, G. S.; Wu, Z.; Tian, C.; Guo, Y.; Dai, S.; Zhan, W., Optimizing the Structural Configuration of Fept-Feox Nanoparticles at the Atomic Scale by Tuning the Post-Synthetic Conditions. Nano Energy 2019, 55, 441-446.
Zhang, S.; Tang, Y.; Nguyen, L.; Zhao, Y.-F.; Wu, Z.; Goh, T.-W.; Liu, J. J.; Li, Y.; Zhu, T.; Huang, W., Catalysis on Singly Dispersed Rh Atoms Anchored on an Inert Support. ACS Catalysis 2018, 8, 110-121.
Polo Garzon, F.; Fung, V.; Liu, X.; Hood, Z. D.; Bickel, E. E.; Bai, L.; Tian, H.; Foo, G. S.; Chi, M.; Jiang, D.-e., Understanding the Impact of Surface Reconstruction of Perovskite Catalysts on Ch4 Activation and Combustion. ACS Catalysis 2018, 8, 10306–10315.
Zhu, W.; Wu, Z.; Foo, G. S.; Gao, X.; Zhou, M.; Liu, B.; Veith, G. M.; Wu, P.; Browning, K. L.; Lee, H. N., Taming Interfacial Electronic Properties of Platinum Nanoparticles on Vacancy-Abundant Boron Nitride Nanosheets for Enhanced Catalysis. Nature communications 2017, 8, 15291.
Zhu, M.; Lai, J.-K.; Tumuluri, U.; Wu, Z.; Wachs, I. E., Nature of Active Sites and Surface Intermediates During Scr of No with Nh3 by Supported V2o5–Wo3/Tio2 Catalysts. Journal of the American Chemical Society 2017, 139, 15624–15627.
Wu, Z.; Cheng, Y.; Tao, F.; Daemen, L.; Foo, G. S.; Nguyen, L.; Zhang, X.; Beste, A.; Ramirez-Cuesta, A. J., Direct Neutron Spectroscopy Observation of Cerium Hydride Species on a Cerium Oxide Catalyst. Journal of the American Chemical Society 2017, 139, 9721-9727.
Tumuluri, U.; Howe, J. D.; Mounfield, W. P.; Li, M.; Chi, M.; Hood, Z. D.; Walton, K. S.; Sholl, D. S.; Dai, S.; Wu, Z., Effect of Surface Structure of Tio2 Nanoparticles on Co2 Adsorption and So2 Resistance. ACS Sustainable Chemistry & Engineering 2017, 5, 9295–9306.
Polo-Garzon, F.; Yang, S.-Z.; Fung, V.; Foo, G. S.; Bickel, E. E.; Chisholm, M. F.; Jiang, D.-e.; Wu, Z., Controlling Reaction Selectivity Via Surface Termination of Perovskite Catalysts. Angewandte Chemie International Edition 2017, 56, 9820–9824.
Foo, G. S.; Hu, G.; Hood, Z. D.; Li, M.; Jiang, D.-e.; Wu, Z., Kinetics and Mechanism of Methanol Conversion over Anatase Titania Nanoshapes. ACS Catalysis 2017, 7, 5345-5356.
