Far out: Fueling the future of space exploration
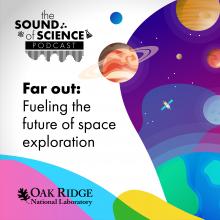
Plutonium-238 is critical to deep space exploration. The special isotope has powered missions that have enabled us to explore the atmosphere of Saturn, see the heart of Pluto and traverse the surface of Mars. But what if missions like those were no longer possible? That's a question NASA faced not so long ago as the U.S. was in short supply of the isotope. The decision was made to restart pu-238 production and Oak Ridge National Laboratory was the place to do it. In this episode you'll hear from the manager of the plutonium program, as well as from the team that makes the cladding to hold the fuel.
Transcript
[THEME MUSIC]
INTRO/VOICES
MCNUTT: It’s pulling the curtain back from all of the unknown.
WHAM: You can’t run out for a repair man. You have to be totally reliable in whatever you put out into space.
GEORGE: I, we, ORNL, we all played a part and it’s cool.
JENNY: Hello everyone and welcome to the Sound of Science, the podcast highlighting the voices behind the breakthroughs at Oak Ridge National Laboratory.
MORGAN/JENNY: We’re your hosts, Morgan McCorkle and Jenny Woodbery.
[VOYAGER SOUNDS]
[SPACEY MUSIC]
MORGAN: Did you hear that? That’s not a problem with our audio. That’s the sound of interstellar space.
JENNY: This was recorded by NASA’s Voyager 1 spacecraft, which crossed over into the interstellar medium in 2012.
MORGAN: Interstellar space is located about 11 billion miles from Earth and is literally defined as the space between the stars. But that doesn’t give you much of an idea of what it really is.
JENNY: Think of our solar system like a bubble. The Sun’s solar winds create a giant bubble around the solar system, called the heliosphere. Once you go past the edge of the bubble – where the Sun’s particles and magnetic field stop affecting its surroundings – you’ve reached interstellar space.
MORGAN: Six years after Voyager 1 reached interstellar space, its sister mission, Voyager 2, arrived.
JENNY: What’s remarkable is that these probes were launched in 1977 and they’re still going strong over 40 years later.
MORGAN: So, what’s the secret to their long life?
JENNY: Plutonium-238.
MORGAN: Plutonium-238, or Pu-238, is a special isotope that NASA uses to fuel deep space missions. As it decays, it emits heat that can be converted into power for spacecraft instrumentation.
JENNY: It has powered missions that have enabled us explore the atmosphere of Saturn, see the heart of Pluto and traverse the surface of Mars.
MORGAN: But what if missions like these were no longer possible?
JENNY: That’s a question NASA faced not so long ago as the viable supply of Pu-238 was quickly dwindling.
MORGAN: The U.S. Department of Energy’s Savannah River Site had been the primary producer of NASA’s Pu-238 supply but it stopped producing the isotope in the late 80s.
JENNY: As the Savannah River supply was starting to age, NASA began supplementing it with material from Russia.
MORGAN: With the quantity and quality of the supply decreasing, it became clear the U.S. would need to address this issue if NASA was going to pursue future deep space missions.
JENNY: This predicament drew the interest of life-long space aficionado Ralph McNutt, who is the chief scientist for space science at the Johns Hopkins University Applied Physics Laboratory.
RALPH MCNUTT: It just turns out that there are some missions in space that you simply can’t do without plutonium-238, and so I decided to cause some trouble, got to talking with people. And one thing led to another and there was a decision made that the U.S. ought to get back into the plutonium-238 business.
MORGAN: Ralph soon found himself co-chairing a report for the National Academies of Sciences, Engineering and Medicine to see how and where this could be done. It was during that time that he visited Oak Ridge National Lab to explore the possibility of starting plutonium production at the High Flux Isotope Reactor, or HFIR.
MCNUTT: I actually came out to Oak Ridge and we got a chance to talk to people here and talk to people about what was going on and what could be done. Really the key to this was the HFIR reactor with the large neutron flux.
JENNY: The unique capabilities of HFIR and the neighboring Radiochemical Engineering Development Center made ORNL an ideal location to restart production.
MORGAN: In 2013, NASA funded DOE’s Office of Nuclear Energy to restart production, and in 2015, the lab produced its first 50 grams of plutonium-238.
JENNY: Let’s fast forward to today. ORNL has now produced 600 grams of plutonium-238 and is continuing to ramp up production.
MORGAN: We talked to Bob Wham, who manages the plutonium-238 program at Oak Ridge, about what it’s like to make this isotope. Beginning with how he’d explain his role at a social gathering.
BOB WHAM: Well the first thing they do is “oh no that’s plutonium,” and you gotta explain it’s not weapons-grade plutonium, it’s plutonium for deep space missions, it doesn’t go boom. This is a special isotope that releases a lot of heat when it decays. And the heat that it releases is converted to electricity, or power, that can be used aboard a deep space mission.
MORGAN: Plutonium has a 90-year half-life, which means half of it will be gone in 90 years. This is why it’s so ideal for powering missions that could last decades – like the Voyagers.
WHAM: You don’t have extension cords. You can’t run out for a repair man. You have to be totally reliable in whatever you put out into space.
JENNY: So just how is this isotope made?
WHAM: So, in order to make plutonium 238 you have to start with a feedstock. And that feedstock is known as neptunium-237.
MORGAN: The process begins at REDC where the neptunium-237 powder is mixed with an aluminum powder. The mixture is pressed into small cylindrical pellets – similar to the size and shape of a pencil eraser.
WHAM: So, when we make 52 of those pellets, we load them into an aluminum tube. And once we load that aluminum tube, we weld that tube shut and do some tests on it to verify the integrity of the tube. And at that point we call it a target.
JENNY: The targets are then irradiated in the High Flux Isotope Reactor for about 50 or 60 days.
MORGAN: The reactor’s fast flow of neutrons enables it to create synthetic isotopes that are very difficult to make like californium, berkelium and of course, plutonium-238.
JENNY: At the end of the cycle, the targets are taken back to REDC where the plutonium is separated from the neptunium.
MORGAN: This work is done in what is called a hot cell. This is a shielded workspace where technicians can safely handle radioactive material by using mechanical arms located behind four feet of leaded glass that’s filled with oil.
JENNY: Once the plutonium is separated from the neptunium, there are still a few steps to get it ready to fuel a spacecraft.
MORGAN: ORNL ships the plutonium-238 in the form of an oxide powder to Los Alamos National Laboratory.
WHAM: Los Alamos processes that plutonium and makes a pellet, and the pellet is about the size of a marshmallow — a large marshmallow. And then that gets encapsulated in the iridium. Once they verify the integrity of those pellets, those get shipped to Idaho National Lab where it can be assembled into the radioisotope power system.
JENNY: The radioisotope thermoelectric generator, or RTG, is the mechanism aboard a space craft that converts heat produced from the plutonium-238 into electricity.
WHAM: So, plutonium 238 gives off enough heat, and it gives out about 2,000 watts for each RTG, and that’s converted into electricity, and it only converts to about 100 or 125 watts of electricity. So, like one of the old incandescent bulbs.
MORGAN: If you’ve seen the movie “The Martian,” you may remember an RTG as the device that kept Matt Damon’s character warm as he traveled on the surface of Mars.
JENNY: If the scenario in the movie were real, the heat from the RTG would have been about the same as a small campfire for the stranded astronaut.
[MUSIC TRANSITION]
MORGAN: The lab is ramping up its production of plutonium-238 and will eventually provide NASA with 1.5 kilograms per year.
WHAM: The RTG takes about 4.5 kilograms of plutonium. So roughly every three years, they’ll be able to fuel a new mission with the plutonium coming from Oak Ridge National Lab.
JENNY: Mars2020 will be the first NASA mission to have a bit of ORNL-produced plutonium on it. And that won’t be the only contribution from ORNL onboard. You may have heard Bob mention that the plutonium fuel in an RTG is encapsulated in iridium – another specialty material produced at the lab.
MORGAN: And that program been going on for decades. In fact, plutonium-238 used on the Voyager missions was contained in iridium made at ORNL. This capsule is called a clad vent set.
EASO GEORGE: The clad vent set is the metal part that protects the plutonium from any accidental scenarios, if there's an accident either during launch or during near Earth orbit. So, you want to have a very strong container that will protect the plutonium from being accidentally released.
MORGAN: That’s Easo George. He holds a Governor’s Chair position at the University of Tennessee and ORNL. Easo has been helping in the development and characterization of alloys used in clad vent sets for nearly three decades.
GEORGE: So, it looks like two big thimbles, if people know what a thimble is. I don't know if the young people know what what a thimble is anymore, but small cups very tiny cups. Tiny espresso cups without the handles and the plutonium is put inside and then the two cups of brought together and welded shut.
JENNY: Making small metal cups sounds easy enough, but it’s actually a pretty complicated process. Iridium can withstand extreme environmental conditions, but it can be a little temperamental to work with.
GEORGE: Iridium is an unusual metal, it has the same crystal structure has more ductile metals like nickel or stainless steel, but in reality, it's a borderline brittle material. So, processing this material requires a lot of skill, very narrow windows of temperature and strain rate over which it can be processed. If you don't do it right, it ends up cracking and so it's a pretty involved process to make these cups.
MORGAN: ORNL has been custom designing the iridium alloy that is used for the clad vent sets since the 1970s. George Ulrich, who manages the iridium program at ORNL, says the material requires a fine-tuned recipe.
GEORGE ULRICH: The alloy itself that we use currently is called DOP 26. DOP is short for dopants, where we're adding 3,000 parts per million – little -- we're not talking percentages or half percent or anything like that. So, 3,000 of the tungsten, 60 parts per million of the thorium, so I mean, this is a little pinch here and a little pinch there.
JENNY: The alloy starts as a powder that goes through a series of detailed processes to turn into a sheet of metal. Circles are cut out of the metal and formed into cups that will hold the fuel. This entire process takes over a year to complete.
MORGAN: Since it’s housing radioactive material, the integrity of the alloy is extremely important.
GEORGE: So, one of the things that we worry about is that if there's an accident either during launch or shortly after launch, that when these generators come back to Earth, and they'll crash into Earth at very high velocity, at very high speeds. And we want to make sure that if such very low probability event but if something like that were to happen, that the iridium would contain the plutonium fuel and prevent it contaminating areas around where it strikes.
JENNY: Easo George took us along to watch how researchers test the performance of the iridium under these kind of extreme conditions.
[GAS GUN SOUND]
GEORGE: So, there's a gas gun that we use, the gas is used to fire a bullet and the bullet then strikes an impact plate. And the impact plate is allowed to move and it then stretches the iridium samples. There's a specific geometry that we test, and we are one of the few places that can do such tests at high strain rates in tension. Many people do these kinds of tests in compression, but since we're interested in ductility, we have to be able to do these in tension, which means we stretch them rather than and push them and Oak Ridge is one of the few places where we can do these kinds of tests at very high temperatures, so we can test them at temperatures up to 1200, 1300 Celsius.
MORGAN: Producing the iridium clad vent sets is a painstaking process. And it has to be done several years before a mission launch so they can be built into the RTG.
JENNY: We asked George Ulrich what it feel like to finally see a mission take off into space?
ULRICH: It is rewarding. About every five years we have a launch. And when we're going to Mars, the neat thing about going to Mars is we have a launch and then six months later, we have a landing and so that's nice. But every so often, we have a launch and then like going to Pluto, we wait nine years before its mission starts, or Cassini going to Saturn, Galileo going to Jupiter. I mean, the launch is rewarding, and I, we, ORNL, and NASA, I mean we all had a part, and it's cool to be part of that.
[MUSIC TRANSITION]
JENNY: Now that ORNL is producing plutonium-238, it’s no longer a question of if NASA can fuel a mission – it’s a question of what to explore next.
RALPH MCNUTT: I’ve been a space cadet since I was a kid. And you know it’s the whole exploration thing. I mean I just I can’t I have a hard time relating to people who can’t be excited about all of this. I mean it’s going to new places, it’s learning new things, it’s sort of pulling the curtain back from all of the unknown. You know you look at the old maps of the world back from not too long after Columbus’s voyage and there were always the edges that was you know “here there be dragons”—the areas that we just didn’t understand, and the speculation about our own place in the universe of course has evolved just remarkably over the last century.
MORGAN: Ralph is eyeing a mission to further pull back the curtain of the mystery that is interstellar space.
MCNUTT: One of the things that has sort of been on the books for a long time within the robotics science part of NASA is the idea of getting a really fast probe out to as far as you can get from the sun, and really trying to understand how that our own home and space really interacts with the interstellar medium. And of course, one of the things that’s happened is that the two Voyagers, Voyager 1 and Voyager 2, have gotten into what we think is the very local interstellar medium, so they’re out there, but they are also ending. We’ve been flying for over 42 years with those spacecrafts. They were designed to last four and a half years, so went way past the warranty.
JENNY: Ralph McNutt says the Voyagers have only scratched the surface of what we can discover about interstellar space and believes now is the time to take a purposeful step into the medium.
MORGAN: He is hoping the mission provisionally known as the Interstellar Probe will be possible in the early 2030s.
MCNUTT: You have to keep reaching out, you have to keep trying, and I keep thinking well perhaps the appropriate quote there is the line from the “Tao Te Ching” by Lao Tzu, that “the thousand-mile journey begins with a single step.” Again, every time we reach out, every time we go to a new place, well we learn something new, and we learn how to do things differently, and that’s what’s called progress.
And let me just say that it’s a good thing Oak Ridge is in this business because a lot of these things that we now think we can do, if it weren’t for this, they certainly would not be possible in the near future.
[MUSIC. TRANSITION]
JENNY: Thank you so much for listening to this episode of the “Sound of Science.” We’d love to hear from you, so please leave a comment or review wherever you get your podcasts.
MORGAN: You can find us on Apple podcasts, Google Play Music or Spotify. And don’t forget to subscribe so you get the newest episode when it’s released.
JENNY: Until next time!
[MUSIC OUTRO]