75 years of science and technology
Although World War II ended in 1945, the study of nuclear energy had just begun. A federal agency was created in 1946 to manage the development of nuclear energy for both military and civilian purposes, and Gen. Leslie Groves handed over the keys for X-10 and its Graphite Reactor to the new Atomic Energy Commission.
The scientists who had worked at X-10—called Clinton Laboratories and briefly Clinton National Laboratory before becoming Oak Ridge National Laboratory in 1948—had big ideas for a nuclear laboratory free from the Manhattan Project’s air of secrecy. The Graphite Reactor’s potential for creating new isotopes and expanding scientific knowledge was too precious a resource to waste.
To help define the lab’s new mission, the AEC needed someone to lead scientific research. On a year’s leave from Princeton University in 1946 and 1947, Eugene Wigner served as ORNL’s research director. During his short time in Oak Ridge, Wigner charted the lab’s peacetime trajectory by establishing a nuclear training school and overseeing the design of a high-flux reactor that could produce powerful neutron fluxes for research and enable scientists to test materials for power reactors. Wigner also recruited Alexander Hollaender to establish the ORNL Biology Division and established a solid-state research group and a division to investigate the effects of radiation on metals.
When Wigner returned to academic life, reactor physicist Alvin Weinberg took his place as research director and later became laboratory director. Weinberg had worked with Wigner on the development of the Chicago Pile and Graphite Reactor, and, like Wigner, he was eager to pursue peaceful uses of nuclear energy. Weinberg had testified before Congress on the topic in 1945 and moved to Oak Ridge to work with Wigner on reactor development. During his nearly 30 years at the laboratory, Weinberg was an important visionary in the new nuclear era (See “Weinberg saves ORNL”).
Nuclear medicine
Isotope production for nuclear medicine was one of the primary applications of the Graphite Reactor after the war. In 1946, ORNL sent the first official shipment of a medical radioisotope, carbon-14, to a hospital—Barnard Free Skin and Cancer Hospital in St. Louis.
The invention of the nuclear reactor enabled the development of an expansive catalog of isotopes with unique properties. By the end of the decade, the lab was producing and distributing more than 50 isotopes created at the Graphite Reactor, generating up to $1 million of revenue for the U.S. government each year.
The advent of reactor-produced isotopes—such as iodine-131 for radiation therapy of hyperthyroidism and phosphorus-32 for imaging tumors and treating cancer—showed that nuclear medicine could radically improve medical diagnosis and treatment. Today, the lab continues to support DOE’s isotope mission through its current nuclear reactor, the High Flux Isotope Reactor. ORNL produces californium-252, used by industry and in cancer treatment, and actinium-227, which is used in treatments of metastatic prostate cancer. With the recent approval of lutetium-177 as a treatment for certain types of pancreatic cancers, ORNL is investigating how to produce this radioisotope with HFIR.
Isotopes also enabled a range of new scientific techniques. Radioactive tracers, made up of short-lived radioisotopes linked to chemical compounds, make it possible to observe specific physical, chemical and biological processes at the molecular level. The ability to track the progress of the compound through a living organism by measuring the decay of the radioisotope has enabled advances in medicine, health physics and organic chemistry.
Health physics
The hazards of radioactivity were not lost on the physicists who studied nuclear energy during the Manhattan Project. Marie Curie had died in 1934 from leukemia resulting from her work with radium, and the effects of X-rays and natural radioactivity had been under scrutiny for decades.
A “health physics” group to study radiation exposure and detection was established at the Metallurgical Laboratory in Chicago in 1942. Led by Ernest Wollan, it would move with Wollan to Oak Ridge before the Manhattan Project was over. This group would also lead the development of “film badges” to monitor worker exposure.
It was clear in 1946 that if nuclear energy were to be the future of power production and scientific research, scientists needed a deeper understanding of the effects of radiation on living organisms. A formal Health Physics Division was established that year, and division staff began monitoring radiation in work areas and the Oak Ridge environment and developing new radiation detection devices, such as dosimeters.
The value of work done at ORNL toward nuclear safety and radiation monitoring is immense. Early textbooks on radiation safety, reference manuals to calculate safe and unsafe exposure levels, and computer models for predicting the transport of radiation through the environment were all developed at ORNL.
To better understand the effects of radiation on mammals, the lab established the Mammalian Genetics Program in 1947. This program would become the foundation for a world-leading genetics laboratory, housed in a facility known as the “Mouse House” for the thousands of mice deliberately exposed to low-dose radiation and later to other carcinogenic and teratogenic substances. In 1952, the mouse studies resulted in guidelines to avoid the increased risks of diagnostic X-rays during early pregnancy that were adopted worldwide (see “The house the Russells built”).
Power reactors—for land, air, and sea
In 1948, ORNL researchers produced electricity from nuclear power for the first time using a toy steam generator connected to a water supply at the Graphite Reactor. Four years later, ORNL researchers sent the first nuclear-generated electricity to the grid from the Homogeneous Reactor Experiment. Although it was the role of industry to provide nuclear power for millions, ORNL and other national labs would work out the foundations of nuclear energy and power generation through many reactor experiments, some of which were one-of-a-kind.
The nuclear navy
The Navy was interested in nuclear power from the beginning and started funding nuclear research during the dizzying breakthrough year of 1939, following the discovery of fission. Researchers at the Naval Research Laboratory even contributed to the Manhattan Project by developing a second diffusion process for enriching uranium: thermal gaseous diffusion, for which the S-50 plant—located at the Oak Ridge K-25 site—was built.
After the war, naval officers visited Clinton Laboratories and enrolled in the Clinton Training School (see “Oak Ridge spreads the nuclear knowledge”). Among these officers was Hyman Rickover, who would become known as the “father of the nuclear navy.” By many accounts, including Weinberg’s memoirs, Rickover could be a rude and difficult character, but his passion for building a nuclear reactor for marine propulsion helped ignite the widespread adoption of nuclear power.
A nuclear reactor would allow submarines to stay under water for almost unlimited periods, greatly expanding the Navy’s reach. After his time at Clinton Laboratories, and particularly after discussions with Weinberg, Rickover recommended in 1950 that the Navy build a pressurized water reactor for submarine propulsion. The design was compact, and water was an abundant, familiar resource.
The first nuclear-powered submarine, the USS Nautilus, went to sea in 1955 and subsequently broke many speed and distance records. By cruising under the Arctic ice pack, it was also the first vessel to cross the North Pole. Following the success of the nuclear submarine, companies looking to build nuclear power reactors for generating commercial electricity also focused on pressurized water reactors, eventually leading to the dominant design for the nuclear power plants that provide 20 percent of U.S. electricity today.
The nuclear aircraft project
Likewise, the Army dreamed of nuclear-powered planes that would not need to land for refueling. In 1946, the Army Air Forces established the Aircraft Nuclear Propulsion program to develop a compact reactor that would power a jet engine. An aircraft reactor was a significant challenge. The reactor needed to be small enough to fit on a plane, encased in shielding to protect passengers, and able to withstand the high temperatures necessary to generate enough power for flight.
After years of experimenting and several innovations, ORNL physicists and engineers built a prototype reactor in 1955 called the “fireball,” a spherical, 60-megawatt reactor that glowed red-hot at 1,500 degrees Celsius. The “fireball” reactor and a previous Aircraft Reactor Experiment prototype successfully demonstrated key components of the ORNL design: a tightly packed honeycomb of beryllium-oxide blocks for a moderator and molten salt coolants that flowed in thin channels of the corrosion-resistant, nickel-based Hastelloy-N. The alloy was developed at the lab in partnership with industry for use at high temperatures and later was commercialized for a range of industrial applications.
Although the two prototypes were a technical success, the AEC began to question the entire enterprise of nuclear-powered flight. Designing a lightweight reactor to operate at such high temperatures while meeting all safety requirements was ultimately viewed as unrealistic.
Over the course of nearly a decade, however, Aircraft Nuclear Propulsion program funding supplied ORNL with several critical research tools, including a powerful particle accelerator and computer, that the lab would leverage to attract new research during the tough times after the aircraft program shut down.
During the height of the Aircraft Nuclear Propulsion project in the 1950s, researchers decided they needed to test possible aircraft reactors and shielding in the air to minimize the scattering of radiation from the reactor by the ground or structures. For this purpose, ORNL built the Tower Shielding Facility, which included four 300-foot towers connected by cables that could hold a reactor and a variety of shield samples up to 200 feet above ground.
From 1954 to 1958, researchers tested the Tower Shielding Reactor-1, which was a smaller version of the Bulk Shielding Reactor—a 500-kilowatt water-cooled reactor encapsulated in a 12-foot-diameter tank. A spherical reactor, the TSR-II, was built in 1958 so researchers could test the spherical design they thought would work best for an aircraft reactor. Eventually the TSR-II operated at 1 megawatt of power.
Despite the end of the nuclear-powered aircraft project, several uses were found for the unique facility—including studies of shielding for space reactors, the effects of radiation on a jet engine, the stability of casks used to transport radioactive fuels (which were dropped from the towers in one dramatic study), and other shielding and radiation projects—until the facility was permanently closed in 1992. The towers are still in place and are visible from the visitor overlook at TVA’s Melton Hill Dam.
The test reactors
The Low-Intensity Test Reactor. ORNL’s Low-Intensity Test Reactor was never meant to be an operational reactor. Instead, it was built as a shell to test the mechanical design of the Materials Test Reactor—a 40-megawatt water-cooled reactor principally designed at ORNL with significant influence from Wigner. Soon after Wigner departed Oak Ridge in 1947 but before the Materials Test Reactor was built, the AEC announced plans to transfer all power reactor projects, including the Materials Test Reactor, to Argonne National Laboratory—the Chicago-based lab that evolved from the Metallurgical Laboratory.
The reactor design called for ordinary (or “light”) water to serve as both a moderator and coolant, with uranium fuel packed between aluminum plates surrounded by beryllium to reflect neutrons. In 1950, Weinberg convinced the AEC that ORNL should add uranium fuel and the beryllium reflector elements to the LITR so they could make measurements to predict and improve the future Materials Test Reactor’s performance. Once the fuel was added to the LITR, the world was introduced to the blue glow known as Cherenkov radiation. The image of the glowing reactor core was published on a 1951 cover of Scientific American and became a popular symbol of nuclear power. (It certainly helped that Weinberg and colleagues sent the magazine the photograph.)
Over its 20 years of operation, the full-scale Materials Test Reactor—which was eventually built at the AEC’s National Reactor Testing Station in Idaho, where it was operated by Argonne—was an important precursor to commercial nuclear power plants and a testbed for nuclear submarine materials. At least part of its success can be attributed to the “poor man’s pile,” as Wigner called the LITR, at Oak Ridge.
The Homogeneous Reactors. Concerned that uranium would become increasingly scarce, the physicists who had worked on the wartime uranium-fueled reactor designs conceived of “breeders”—reactors that used fission to produce more fuel than the reactors consumed.
In 1954, President Dwight D. Eisenhower opened the floodgates to nuclear commercialization through an amendment to the Atomic Energy Act as part of his “Atoms for Peace” policy. Private industry followed the example of the Navy and focused on water-cooled reactors. It made sense for the national laboratories to develop a more “long-term” solution to nuclear power; Weinberg and others in the mid-20th century believed breeder reactors could meet that long-term need.
Meanwhile, Britain, which had fallen behind in reactor development during World War II, built a series of gas-cooled reactors in the early 1950s. In reaction, the AEC tasked its laboratories with researching different gas-cooled designs, and ORNL was appointed the task of researching homogeneous reactors.
As the name suggests, homogeneous reactors rely on the same solution for fuel, moderator and coolant. This mixed solution circulates continuously, so a homogeneous reactor does not need to be stopped to discard spent fuel. However, because the fuel solution circulates through the entire reactor, not just the core, workers must contend with more radioactive components, which can make maintenance tedious and costly.
In 1952, ORNL built the Homogeneous Reactor Experiment to demonstrate that a homogeneous reactor was a feasible design for producing power. The experiment would turn out to be one of the more heart-pounding—literally. Some of the pumps used to draw waste gas from the HRE “made a horrible racket that reverberated through the control room of the reactor and almost gave us heart failure, because we feared the diaphragm would burst,” Weinberg wrote in his book "The First Nuclear Era."
Despite the noise, the reactor proved to be remarkably self-regulating, able to maintain a natural balance between temperature, pressure and power. This discovery, along with others, proved the project a success. However, more research was needed to solve remaining technical challenges, including the frustrating maintenance of radioactive parts and the tendency of the reactor’s many pumps and valves to leak from fuel buildup.
In 1957, the lab built a larger homogeneous reactor, called the Homogeneous Reactor Test, which reached its full power of 5 megawatts in 1958. At the time, the HRT beat the record for the longest continuous reactor operation at 105 days, but by 1959 corrosion caused a hole in the tank, leading to reactor shutdown—and tanking the aqueous homogeneous reactor project, as well.
The Molten Salt Reactor Experiment. Although the nuclear-powered aircraft project was abandoned in 1961, the molten salt fuel developed to power a reactor at extremely high temperatures had been a technical success. In 1960, ORNL began designing the Molten Salt Reactor Experiment, in which a fluoride-mix fuel ran along channels in a graphite core.
All of the pumps and components that touched the radioactive molten salt fuel were built from Hastelloy-N. Learning from the laborious maintenance required for the homogeneous reactors, the ORNL development team installed additional shielding around the reactor and carefully laid out the components so they could be accessed using long-handled tools.
Perhaps the most significant achievement of the MSRE occurred in 1966 when fissile uranium-233 fuel was added to the reactor fuel—making the MSRE the first reactor to operate on uranium-233. Glenn Seaborg, who had codiscovered uranium-233 during the early days of the Manhattan Project, came to ORNL to start up the reactor for uranium-233 operation.
Years earlier, Wigner had proposed a thermal breeder reactor using a uranium-233 and thorium-232 fuel cycle. In MSRE, Weinberg saw a stepping stone to building a thermal breeder reactor, but the AEC shut down the molten salt program in 1973 in favor of another type of breeder reactor: the fast breeder. ORNL’s expertise in molten salt chemistry has become newly relevant as the technology is considered for cooling conventional fission reactors and as concepts for new liquid-fueled reactors are developed.
Reactors for research: Reactor design and radiation safety
The Bulk Shielding Reactor. Until the development of the “swimming pool” reactors, as they would be named because of their open tanks of water, researchers relied on either lower-power reactors or small channels in higher-power reactors for conducting experiments. Testing the effects of radiation on bulky shielding materials was difficult. After conducting shielding tests for nuclear Navy projects including the Nautilus, ORNL’s Everett Blizard proposed submerging a reactor vessel in a large tank of water to allow room for testing different material configurations.
In 1950, the lab converted the Materials Test Reactor design to a swimming pool reactor: the 2‑megawatt Bulk Shielding Reactor. At the BSR facility, two bridges over a 20-by-40-foot pool of water enabled engineers on one bridge to move the reactor vessel around the pool with a crane while researchers worked on experiments from the other bridge. A small reactor assembly, known as the Pool Critical Assembly, was stationed in the corner of the tank for small-scale experiments. The BSR’s simple design and versatility supported a broad range of radiation and materials research for more than 30 years, and the design was replicated in about 100 research reactors around the world.
The Health Physics Research Reactor. From 1963 to 1987, the lab operated a fast-burst reactor that could produce pulses of radiation for health physics research. By adjusting the reactor’s pulse mode, researchers could simulate a range of radioactive conditions over 15 orders of magnitude (up to 100,000 megawatts). This versatility enabled them to better study radiation’s impact on the human body and other organisms and develop improved dosimeters for measuring radiation exposure. Studies using the reactor to irradiate the “organs” of human-simulating phantoms contributed significantly to the establishment of safe exposure limits.
Reactors for research: Isotopes and neutrons
Oak Ridge Research Reactor. In 1958, after more than a decade, ORNL researchers finally got a high-flux nuclear reactor like the Materials Test Reactor they once hoped for. Similar to the Materials Test Reactor, the Oak Ridge Research Reactor was a 30-megawatt water-cooled reactor. Like the BSR, it was adapted to include a water tank that enhanced access for research. The tank also prompted the design of an “observation deck,” and an “SS ORR” life preserver was hung on the side. The facility was a popular draw for politicians and dignitaries, including three future U.S. presidents and foreign royalty.
The ORR operated for almost 30 years and was an important tool for advancing American science, particularly in the fields of isotope production and neutron science (see “Making the most of neutrons”). At the ORR, scientists confirmed the electron-neutrino theory of beta decay (which introduced an imbalance in the Standard Model that occupies particle physicists to this day) and conducted strings of studies on the properties of rare earth elements and crystals, among many other achievements.
High Flux Isotope Reactor. In 1965, ORNL would get another high-flux reactor and the most intense neutron source for research in the world. HFIR was built to produce superheavy elements. A Transuranium Processing Plant, now part of the Radiochemical Engineering Development Center, was built next door to recover the superheavy elements and radioisotopes produced at HFIR.
Although the AEC approved HFIR as an isotope production reactor, Weinberg—always looking for ways to amplify and diversify the lab’s research capabilities—saw an opportunity to expand neutron science research as well. He managed to get approval for ORNL to add four beam holes for neutron research. The reactor became an early version of a user facility, introducing scientists from many disciplines to neutrons as tools for studying materials, through a collaboration with the National Science Foundation known as the National Center for Small-Angle Scattering Research, which also provided access to X-ray scattering resources. Today, HFIR is ORNL’s only remaining nuclear reactor and is still a world-leading resource for materials irradiation, isotope production and neutron science.
As the commercial nuclear power industry developed, the lab, under Weinberg’s guidance, turned its tools and expertise to new challenges facing the nation, including growing demands for environmental protection and energy conservation, but the lab’s roughly 20 years of involvement in reactor research and development led to many achievements in materials, chemistry and the physical sciences that would enable further advances during the next quarter-century.
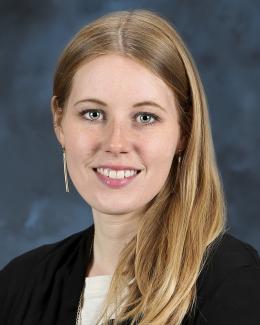