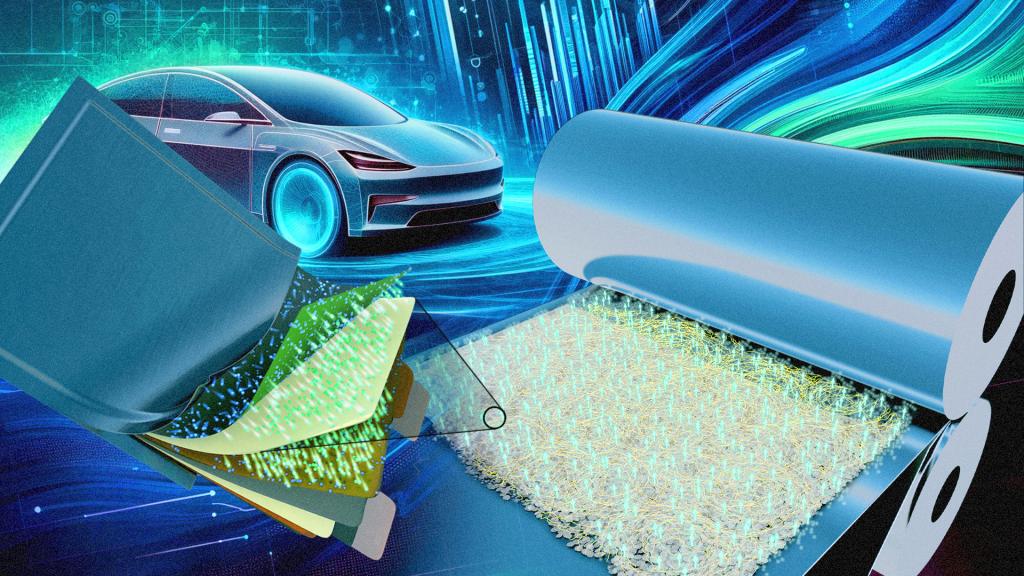
Using a polymer to make a strong yet springy thin film, scientists led by the Department of Energy’s Oak Ridge National Laboratory are speeding the arrival of next-generation solid-state batteries. This effort advances the development of electric vehicle power enabled by flexible, durable sheets of solid-state electrolytes.
The sheets may allow scalable production of future solid-state batteries with higher energy density electrodes. By separating negative and positive electrodes, they would prevent dangerous electrical shorts while providing high-conduction paths for ion movement. These achievements foreshadow greater safety, performance and energy density compared to current batteries that use liquid electrolytes, which are flammable, chemically reactive, thermally unstable and prone to leakage.
“Our achievement could at least double energy storage to 500 watt-hours per kilogram,” said ORNL’s Guang Yang. “The major motivation to develop solid-state electrolyte membranes that are 30 micrometers or thinner was to pack more energy into lithium-ion batteries so your electric vehicles, laptops and cell phones can run much longer before needing to recharge.”
The work, published in ACS Energy Letters, improved on a prior ORNL invention by optimizing the polymer binder for use with sulfide solid-state electrolytes. It is part of ongoing efforts that establish protocols for selecting and processing materials.
The goal of this study was to find the “Goldilocks” spot — a film thickness just right for supporting both ion conduction and structural strength.
Current solid-state electrolytes use a plastic polymer that conducts ions, but their conductivity is much lower than that of liquid electrolytes. Sometimes, polymer electrolytes incorporate liquid electrolytes to improve performance.
Sulfide solid-state electrolyte has ionic conductivity comparable to that of the liquid electrolyte currently used in lithium-ion batteries. “It’s very appealing,” Yang said. “The sulfide compounds create a conducting path that allows lithium to move back and forth during the charge/discharge process.”
The researchers discovered that the polymer binder’s molecular weight is crucial for creating durable solid-state-electrolyte films. Films made with lightweight binders, which have shorter polymer chains, lack the strength to stay in contact with the electrolytic material. By contrast, films made with heavier binders, which have longer polymer chains, have greater structural integrity. Additionally, it takes less long-chain binder to make a good ion-conducting film.
“We want to minimize the polymer binder because it does not conduct ions,” Yang said. “The binder’s only function is to lock the electrolyte particles into the film. Using more binder improves the film’s quality but reduces ion conduction. Conversely, using less binder enhances ion conduction but compromises film quality.“
Yang designed the study’s experiments and oversaw the project, collaborating with Jagjit Nanda, the executive director of the SLAC Stanford Battery Center and a Battelle Distinguished Inventor. Yang was recently recognized by DOE’s Advanced Research Projects Agency-Energy as a scientist likely to succeed in converting innovative ideas into impactful technologies.
Anna Mills, a former graduate student at Florida A&M University-Florida State University College of Engineering, focused on nanomaterial synthesis. She tested thin films using electrochemical impedance spectroscopy and made critical current density measurements. Daniel Hallinan from Florida State provided advice on polymer physics. Ella Williams, a summer intern from Freed-Hardeman University, helped with electrochemical cell fabrication and evaluations.
At the Center for Nanophase Materials Sciences, a DOE Office of Science user facility at ORNL, Yi-Feng Su and Wan-Yu Tsai conducted scanning electron microscopy and energy-dispersive X-ray spectroscopy to characterize the elemental composition and microscopic structure of the thin film. Sergiy Kalnaus, also from ORNL, used nanoindentation to measure local stress and strain on its surface and applied theory to understand the results.
Xueli Zheng and Swetha Vaidyanathan, both of SLAC National Acceleratory Laboratory, performed measurements at the Stanford Synchrotron Radiation Lightsource to reveal the morphology of cathode particles.
These advanced characterization techniques were crucial for examining the intricate details of the sulfide solid-state electrolyte sheet. “By understanding these details, we were able to enhance the electrolyte's ability to conduct ions effectively and maintain its stability,” Yang said. “This detailed analysis is vital for developing more reliable and efficient solid-state batteries.”
The scientists are expanding the capabilities of their 7,000 square feet of ORNL lab space by establishing low-humidity areas dedicated for research with sulfides, which tend to contaminate other materials. “To address this, we need dedicated glove boxes in our chemistry lab,” Yang said. “It can be challenging in many settings to allocate resources for such specialized equipment. At ORNL, we have eight glove boxes specifically for this work.”
The team will build a device that can integrate a thin film into next-generation negative and positive electrodes to test it under practical battery conditions. Then they will partner with researchers in industry, academia and government to develop and test the film in other devices.
”This work is ideally suited for the capabilities available at a national lab,” Yang said, praising teams of diverse experts with access to valuable materials, characterization tools and dedicated facilities.
This research was sponsored by the DOE Office of Energy Efficiency and Renewable Energy’s Vehicle Technologies Office.
UT-Battelle manages ORNL for DOE’s Office of Science. The single largest supporter of basic research in the physical sciences in the United States, the Office of Science is working to address some of the most pressing challenges of our time. For more information, please visit energy.gov/science. — Dawn Levy