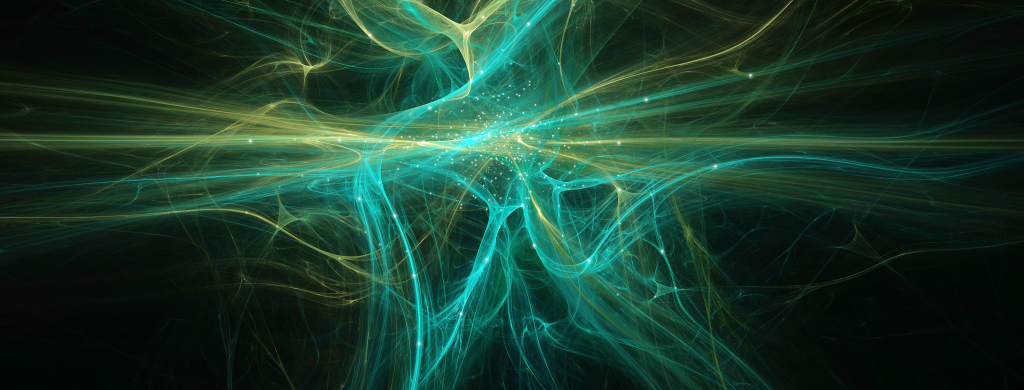
Researchers simulated a key quantum state at one of the largest scales reported, with support from the Quantum Computing User Program, or QCUP, at the Department of Energy’s Oak Ridge National Laboratory.
The techniques used by the team could help develop quantum simulation capabilities for the next generation of quantum computers.
The study used Quantinuum’s H1-1 computer to model a quantum version of a classical mathematical model that tracks how a disease spreads. Time on the computer was provided by QCUP, part of the Oak Ridge Leadership Computing Facility, which awards time on privately owned quantum processors around the country to support research projects.
The model used quantum bits, or qubits, to simulate the transition between active states, such as infection, and inactive states, such as death or recovery.
“The goal of this study was to work toward building capabilities on a quantum computer to solve this problem and others like it that are hard to calculate on conventional computers,” said Andrew Potter, a co-author of the study and assistant professor of physics at the University of British Columbia in Vancouver. “This experiment models trying to steer a quantum system toward a particular state, while competing with the quantum fluctuations away from this state. There’s a transition point where these competing effects exactly balance. That point separates a phase where the steering succeeds and where it fails.”
The farther the system moves out of equilibrium, the more likely classical versions of the model will break down because of the size and complexity of the equations. The research team sought to use quantum computing to model those dynamics.
Classical computers store information in bits equal to either 0 or 1. In other words, a classical bit, like a light switch, exists in one of two states: on or off. That binary dynamic doesn’t necessarily fit modeling transitional states such as those studied in the disease model.
Quantum computing uses the laws of quantum mechanics to store information in qubits, the quantum equivalent of bits. Qubits can exist in more than one state simultaneously via quantum superposition, which allows qubits to carry more information than classical bits.
In quantum superposition, a qubit can exist in two states at the same time, similar to a spinning coin — neither heads nor tails for the coin, neither one frequency nor the other for the qubit. Measuring the value of the qubit determines the probability of measuring either of the two possible values, similar to stopping the coin on heads or tails. That dynamic allows for a wider range of possible values that could be used to study complex questions like transitional states.
Researchers hope those possibilities will drive a quantum revolution that sees quantum computers surpass classical machines in speed and power. But the qubits used by current quantum machines tend to degrade easily. That decay causes high error rates that can muddle results from any model larger than a test problem.
Potter and his colleagues obtained time via QCUP on the Quantinuum computer, which uses trapped ions as qubits. They measured circuits, or quantum gates, throughout the run and used a technique known as qubit recycling to eliminate degraded qubits.
“We used the quantum processor to simulate a system where active qubits have the ability to activate neighboring qubits or become inactive,” Potter said. “By monitoring the system in real time at each step and testing as we go, we could detect the likelihood that performing a quantum gate on a qubit could affect the state of a qubit and, if not, remove it from the calculation. This way we avoid the chance for errors to creep in.”
The team determined they could use their approach on 20 qubits to hold errors down and simulate a quantum system nearly four times that size. They estimated at 70 qubits their approach could equal or surpass a classical computer’s capabilities.
“This is the first time the approach has been used for a system this size,” Potter said.
Next steps include applying qubit recycling to quantum problems, such as simulating properties of materials and calculating their lowest energy states, or quantum ground states.
Support for this research came from the DOE Office of Science’s Advanced Scientific Computing Research program and from the National Science Foundation. The OLCF is a DOE Office of Science user facility at ORNL.
UT-Battelle manages ORNL for DOE’s Office of Science, the single largest supporter of basic research in the physical sciences in the United States. DOE’s Office of Science is working to address some of the most pressing challenges of our time. For more information, visit https://energy.gov/science. — Matt Lakin
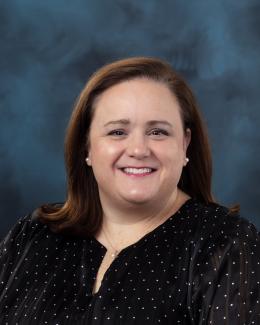