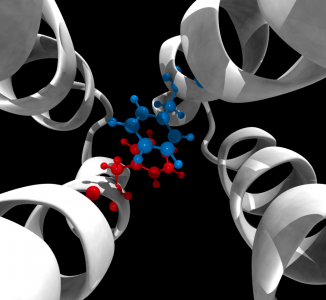
Petaflop computing simulates signaling in a receptor complex of 400,000 atoms
Scientists want to manipulate cell behavior for a lot of reasons. If they can control cell functions such as movement and development, they can cripple cells and pathogens that are causing disease in the body.
Using computational molecular dynamics simulations, researchers at Oak Ridge National Laboratory(ORNL) and the University of Tennessee (UT)–ORNL Joint Institute for Computational Sciences (JICS) have discovered a molecular “switch” in a receptor that controls cell behavior. The team is currently expanding these simulations on the 27-petaflop CPU–GPU machine Titan—the nation’s most powerful supercomputer, managed by the Oak Ridge Leadership Computing Facility at ORNL.
Researchers discovered the molecular switch by simulating 140,000 atoms that make up the signaling part of the Tsr chemoreceptor dimer that controls motility in E. coli. A dimer is a system of two identical receptor molecules. Like other receptors, Tsr spans the cell membrane, communicating to proteins inside the cell in order to respond to threats or opportunities in the environment. Exactly how receptors send signals to neighboring proteins is a critical, unanswered question in molecular cell biology.
The results, published in Nature Communications, directly address this question and stand apart from previous research because of the computational power applied to the problem.
The moving picture show
Parallel computing, which performs many computations simultaneously, made it possible for researchers to simulate such a large molecular system long enough to identify the specific amino acids responsible for protein signaling.
“When we say proteins transmit signals, in most cases we don’t really know what this means in terms of their conformational changes,” said Igor Zhulin, distinguished R&D staff member in the ORNL Computer Science and Mathematics Division , joint professor in the UT Department of Microbiology, and JICS joint faculty member. “For decades proteins have been viewed as static molecules, and almost everything we know about them comes from static images, such as those produced with x-ray crystallography. But signaling is a dynamic process, which is difficult to fully understand using only snapshots.”
To move beyond such snapshots, the team led by Zhulin and Jerome Baudry, assistant professor in the UT Department of Biochemistry and Cellular and Molecular Biology and the UT–ORNL Center for Molecular Biophysics, used a specialty parallel computer called Anton, built by D.E. Shaw Research, that performs speedy molecular dynamics calculations. With Anton the team was able to determine that the seemingly erratic flipping conformations of a single pair of phenylalanine amino acids called Phe396 at the tip of the chemoreceptor were in fact acting as a receptor switch, impacting the shape of the entire chemoreceptor.
“To our knowledge, this is the first time this switch has been described,” said Davi Ortega, lead author and postdoctoral fellow in Zhulin’s lab.
The Phe396 pair is restless, always flipping 180 degrees back and forth relative to the receptor, but researchers found a clear pattern. When the receptor is in signal-on mode, the switch spends more time in the “on” position. When the receptor is in signal-off mode, the switch spends more time in the “off” position.
“It is like a crazy light switch. When you switch it on to light up your room, it occasionally flips down giving you moments of darkness, and when you switch it off to go to sleep, it occasionally goes up flashing,” Zhulin said.
To determine how important this switch is across biological kingdoms, the team compared thousands of chemoreceptor sequences from all available microbial genomes. Remarkably, the Phe396 amino acid was present in all of them, indicating it is likely the switch has existed throughout more than 2 billion years of microbial evolution.
“This amino acid is irreplaceable,” Zhulin said. “When our collaborators at the University of Utah led by John Parkinson substituted phenylalanine in this position with any of the other 19 amino acids, the mutant receptors were all defective.”
Phenylalanine pairs capable of forming such a molecular switch are also present in many other signaling proteins, including receptors in human cells, making it an attractive target for drug design and biotechnology applications.
“Ninety percent of all medical drugs are directed against receptors or pathways controlled by receptors,” Zhulin said. “If we understand how receptors switch on and off, then we can control their actions with new or improved medicines.”
Not quite as large as life
Although researchers were able to simulate a detailed, dynamic Tsr E. coli chemoreceptor dimer on Anton, they do not yet have the entire picture. The dimer does not work alone.
Dimers are grouped in threes to form trimers of dimers—the next rung in the complex signaling array of hundreds of receptor molecules that operate in concert. With a dimer simulation in hand, the team’s next logical step is simulating a trimer of dimers. By simulating a trimer, researchers expect they can learn more about how the Phe396-mediated signal is amplified across neighboring proteins.
But the trimer simulation requires modeling almost 400,000 atoms with increasingly complex physics calculations as the system gets larger. Additionally, to gather statistical significance, researchers must run longer simulations.
“Usually molecular dynamics simulations like these run for about 200 nanoseconds,” Zhulin said. “But in order to see enough changes to explain the signaling mechanism, we need to run the system for at least 2 microseconds. While it still sounds like an incredibly short period of time, we call it physiologically relevant because a lot of things can happen within a protein molecule during this period.”
Longer simulations were no problem on Anton—as long as there involved only 140,000 atoms.
If the next step requires building a simulation system with 10 times as many time steps as conventional molecular dynamics simulations and nearly 400,000 atoms, the group will need a lot of computational capital. Using Titan the researchers ran a preliminary simulation to determine that they would need millions of processing hours on a petaflop machine capable of quadrillions of calculations per second. The machine would also have to operate fast enough to make the study feasible.
“Anton is an exceptional machine, but its hardware limitations won’t permit the simulation of such a large system,” Ortega said.
All roads led back to Titan.
“Resources available on Titan are needed to simulate our system at the level of complexity that actually takes place in the cell,” Baudry said. “In addition to its fundamental interest, this work exemplifies the growing importance of numerical experiments in biology.”
Titan’s GPUs are highly parallel, capable of hurtling through repetitive calculations such as those modeling the large system of atoms under a vast array of configurations in the trimer of dimers simulation.
“We have tested and confirmed that the trimer of dimers system will fit Titan, and we will begin to see how the signal propagates across chemoreceptors,” Zhulin said. “We think this will start to explain how signals are being amplified by these remarkable molecular machines.”
While the team is currently running a trimer of dimers simulation on Titan, it is also exploring how much computational power it will take to simulate even larger receptor complexes than a single trimer. While these kinds of simulations lie farther in the future, Ortega said, systems like Titan are making planning for them possible