Images of the 3-D printed “Frankenstein design” collimator show the “scars” where the individual parts are joined, which are clearly visible at right. Credit: Genevieve Martin/ORNL, U.S. Dept. of Energy
The time-tested strategy of divide and conquer took on a new, high-tech meaning during neutron experiments by scientists at the Department of Energy’s Oak Ridge National Laboratory. They discovered that the problems they faced while attempting to 3-D print a one-piece collimator could be solved by instead developing a “Frankenstein design” involving multiple body parts – and some rather obvious scars.
Collimators are important components used in neutron scattering. Similar to X-rays, neutrons are used to study energy and matter at the atomic scale. Neutron collimators can be thought of as funnels that help guide neutrons toward a detector after they interact with experimental sample materials. These funnels primarily serve to reduce the number of stray neutrons that interfere with data collection, for example, neutrons that scatter off sample holders, or from other apparatuses used in the experiment such as high-pressure cells.
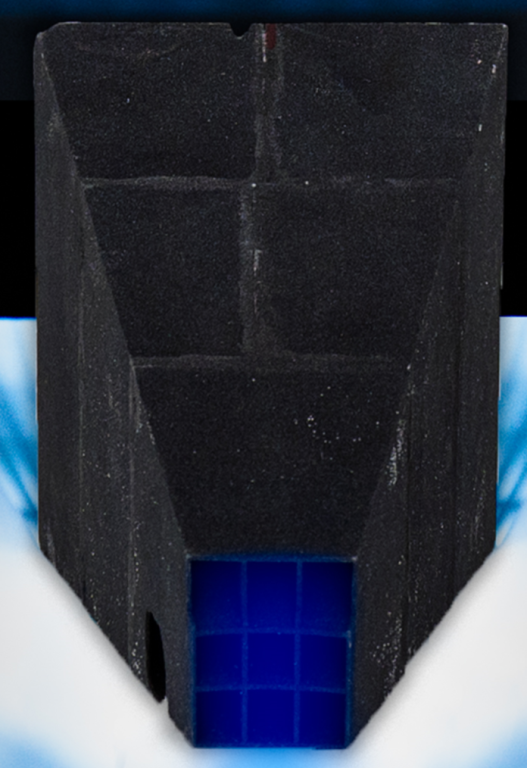
During this process, most of the unwanted neutrons, those scattering from features other than the sample, enter channels inside the collimators at odd angles and are absorbed by channel walls, also referred to as blades. The blades act like the gutters on a bowling lane, which capture bowling balls that are not headed toward the pins.
“The research trend toward using smaller samples of materials in more complex environments results in a larger number of neutrons that did not interact with the sample and are not scattering from the sample,” said Fahima Islam, the study’s lead author and a neutronics scientist at ORNL’s Spallation Neutron Source, or SNS. “These unwanted neutrons produce undesirable signatures in the data, which is why we were working to produce a 3-D printed collimator that could be custom designed to filter out these unwelcome background features during different types of neutron scattering experiments.”
The team collaborated with experts at ORNL’s Manufacturing Demonstration Facility, or MDF, to use a 3-D printing method called binder jetting. This additive manufacturing process builds parts and tools from powdered materials. Similar to printing on paper, the precision process builds the part layer by layer, based on a digital design, until the object is complete.
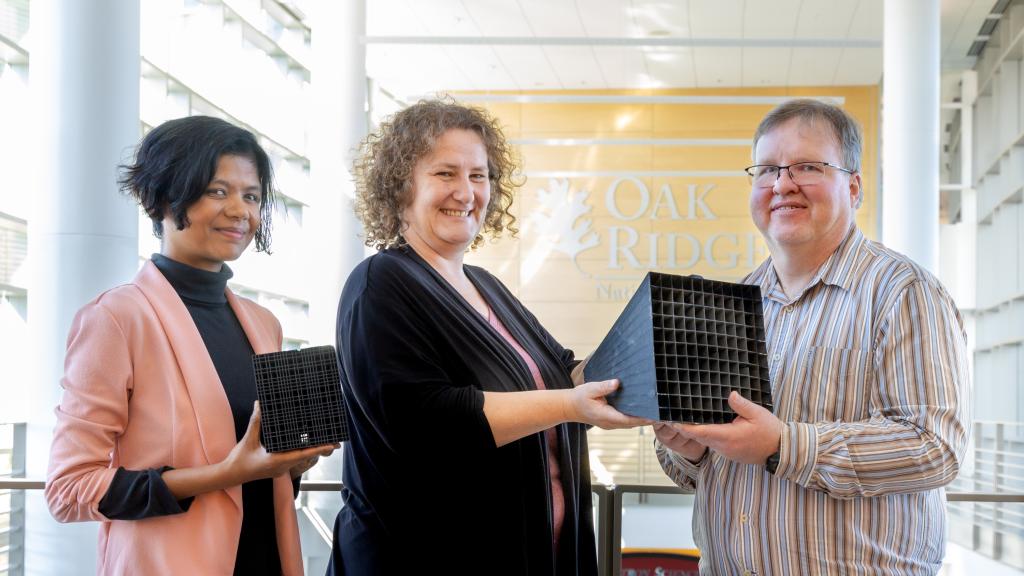
Bianca Haberl and Garrett Granroth. Credit: Genevieve Martin/ORNL, U.S. Dept. of Energy.
One hurdle facing the team involved scaling up the size of the printed collimator while maintaining the accuracy of the finished product. A large collimator was needed to capture a higher number of neutrons scattering from the sample and the complex pressure cell chosen for the test. In a pressurized environment, the sample is encased within a nontransparent sample container, which causes a significant number of unwanted neutrons to scatter strongly in a way that can dominate the weaker data signal that scientists are searching for.
“To demonstrate the viability of using custom-built, 3-D printed collimators, we decided to use a very small sample contained in a diamond anvil cell — a high-pressure chamber that uses diamonds to squeeze materials. Some of these cells are so complex and strong that they are capable of producing pressures approaching those at the center of the Earth,” said Bianca Haberl, the study’s corresponding author and a neutron scattering scientist at SNS. “In fact, high-pressure cells are some of the most complex environments used in neutron experiments, so it is a real challenge to filter out the huge amount of unwanted cell scatter they produce.”
The scientific principles for designing collimators are generally well understood, so the team’s first attempt at 3-D printing a collimator for such a small sample involved simply scaling up the printed part size while retaining the continuous, front-to-back blades that form the channels. The binder jet 3-D printer enabled printing the one-piece version at dimensions of about 12 by 9 by 9 inches, which maximized the capacity to steer neutrons to the detector while still fitting into the instrument.
Unfortunately, the complexities in scaling up the 3-D printing process impaired the precision of the printed part to such a degree it was not suitable to use on the beamline.
“Simply scaling up the print as one large part with continuous blades was clearly not feasible without further optimizing the printing process,” said Garrett Granroth, a co-author and neutron scattering scientist at SNS. “A new concept was subsequently developed to print multiple smaller parts and then manually assemble them into a complete collimator. The main reason for using smaller pieces is that the cracking observed in the single-part design was primarily due to variations in the material’s contraction rate during the curing and cooling process. By reducing their overall size, the individual parts cooled more uniformly.”
An alternate-blade design with progressively tighter blades, from the end facing the sample to the end facing the detector, was used instead. This configuration allowed for a higher density of blades with reduced channel sizes and avoided some size-related 3-D printing limitations. By ensuring the blades did not cross a boundary between the individual parts, the design was less sensitive to misalignment between the pieces during assembly.
Employing this approach, the team optimized the collimator performance by simulating the entire experiment using advanced computational methods developed for the project. The simulation produced a design that could go directly to production without additional engineering.
The 3-D printed, alternate-blade collimator was assessed for performance on SNAP, the Spallation Neutron and Pressure beamline, a dedicated high-pressure neutron diffractometer. Experiments revealed an extreme sensitivity to the collimator’s alignment, emphasizing the necessity for ultrahigh precision in collimator manufacturing and positioning on the beamline.
Once the collimator was precisely aligned, it enabled the desired increase in the relative sample signal over the cell scatter, proving the concept. The scientists also identified areas for future refinement, including further enhancements through more stringent manufacturing quality control and improved alignment. By combining modeling and advanced manufacturing, the study identified a new means of customizing neutron scattering instrumentation and advancing neutron science.
SNS is a DOE Office of Science user facility. The MDF, supported by DOE’s Advanced Materials and Manufacturing Technologies Office, is a nationwide consortium of collaborators working with ORNL to innovate, inspire and catalyze the transformation of U.S. manufacturing.
UT-Battelle manages ORNL for DOE’s Office of Science, the single largest supporter of basic research in the physical sciences in the United States. The Office of Science is working to address some of the most pressing challenges of our time. For more information, please visit energy.gov/science. — Paul Boisvert
