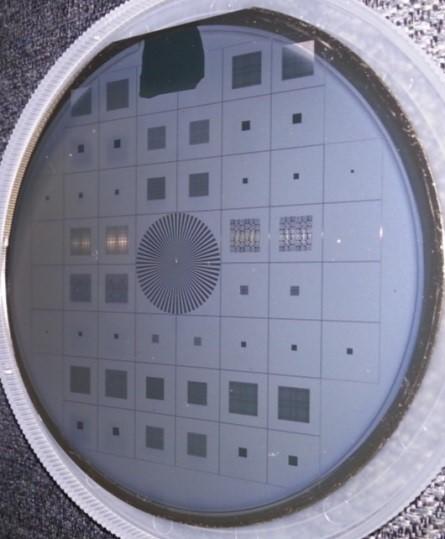
Neutron scattering studies at sites such as ORNL’s High Flux Isotope Reactor (HFIR), a DOE Office of Science User Facility, provide researchers with a wealth of information about the positions, motions, and magnetic properties of solids. With neutron scattering—as with any type of imaging—the higher the resolution, the better and clearer the results.
Researchers at ORNL have spent the last 5 years developing a higher-resolution system that they tested on HFIR’s CG-1D imaging instrument. Philip Bingham, a group leader in EESD’s Electrical and Electronics Systems Research Division, led the early career project to develop a neutron coded aperture method for high-resolution neutron imaging.
Neutron imaging typically uses a large neutron source—millimeters in diameter—to increase neutron flux to a level that allows reasonable exposure times, according to Bingham. The large neutron source requires that objects be placed close to the detector to prevent image blurring. In this mode, resolution is limited to the detector resolution, which cannot meet the needs of many scientific applications. Moving the object closer to the source magnifies the image on the detector, but blurring from the source limits resolution to the size of the neutron source.
“If the source size were smaller, magnification could be used to overcome detector resolution limits, but exposure times would increase,” Bingham said. “It would be going from taking an image in a few minutes to taking an image in a few months.”
Drawing on the idea of a smaller neutron source, Bingham’s team developed coded aperture masks, or wafers containing many small holes (apertures) in a known—or coded—pattern. The mask, which is square, is placed in front of a large neutron source, and the neutrons can pass only through the holes; the result is akin to many small neutron sources providing the desired resolution.
“But now there are a bunch of overlapping images,” Bingham said. “The image that comes out of the system is a blur. However, we know what made that image, and we know where all those rays came from, so we can do a reconstruction to produce an image that looks as if it were captured by a single hole.”
Hector Santos-Villalobos led the team’s efforts to develop software to reconstruct the image using iterative techniques that incorporate detailed mathematical descriptions of the imaging instrument, including the mask.
“We have demonstrated this technique at HFIR, but there are still technical challenges to solve before this technique will see regular use. The mask isn’t thick enough, so neutrons are coming through blocked areas, adding noise to the image,” Bingham said.
Fortunately, having new and different masks created is convenient—Nick Lavrik and his team in the Nanofabrication Research Laboratory at ORNL’s Center for Nanophase Materials Sciences make the masks, which are quartz wafers patterned with gadolinium. Currently the best masks that the team has produced are about as thick as the diameter of the pinholes, or a ratio of about 1:1, and the smallest hole pattern successfully tested at HFIR contains holes measuring 10 microns by 10 microns. --Wendy Hames